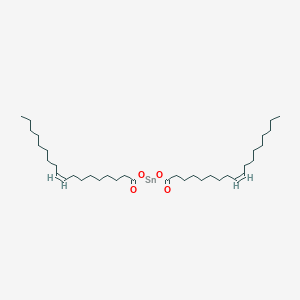
Tin (II) oleate
- Click on QUICK INQUIRY to receive a quote from our team of experts.
- With the quality product at a COMPETITIVE price, you can focus more on your research.
Overview
Description
Tin (II) oleate, also known as stannous oleate, is an organotin compound where tin is in the +2 oxidation state. This compound is formed by the reaction of tin with oleic acid, a fatty acid commonly found in various animal and vegetable fats and oils. This compound is known for its applications in catalysis, particularly in the production of silicones and polyurethanes .
Preparation Methods
Synthetic Routes and Reaction Conditions: Tin (II) oleate can be synthesized through the direct reaction of tin (II) chloride with oleic acid. The reaction typically involves heating the mixture to facilitate the formation of the this compound compound. The general reaction is as follows:
SnCl2+2C18H34O2→Sn(C18H33O2)2+2HCl
Industrial Production Methods: In industrial settings, this compound is often produced by reacting tin metal or tin oxides with oleic acid under controlled conditions. This process may involve the use of a promoter and an oxygen-containing gas to facilitate the reaction .
Types of Reactions:
Oxidation: this compound can undergo oxidation to form tin (IV) compounds.
Reduction: It can be reduced back to elemental tin under specific conditions.
Substitution: this compound can participate in substitution reactions where the oleate ligands are replaced by other ligands.
Common Reagents and Conditions:
Oxidizing Agents: Oxygen or other oxidizing agents can be used to oxidize this compound.
Reducing Agents: Elemental tin or other reducing agents can be used to reduce this compound.
Substitution Reactions: Various ligands can be introduced to replace the oleate ligands under appropriate conditions.
Major Products:
Oxidation: Tin (IV) oleate or other tin (IV) compounds.
Reduction: Elemental tin.
Substitution: New organotin compounds with different ligands
Scientific Research Applications
Tin (II) oleate has a wide range of applications in scientific research and industry:
Chemistry: Used as a catalyst in the production of silicones and polyurethanes.
Medicine: Utilized in the formulation of certain medical-grade silicones.
Industry: Acts as a lubricant additive to reduce friction and wear in machinery.
Mechanism of Action
The mechanism by which tin (II) oleate exerts its effects is primarily through its role as a catalyst. The tin atom in this compound can coordinate with various functional groups, such as isocyanates and alcohols, facilitating reactions like polymerization and esterification. This coordination ability enhances the reactivity of the functional groups, leading to more efficient chemical processes .
Comparison with Similar Compounds
Tin (II) octoate: Another organotin compound used as a catalyst in similar applications.
Dioctyltin dilaurate: Used in the production of silicones and polyurethanes.
Dibutyltin dilaurate: Commonly used in the production of polyurethanes and as a stabilizer in PVC
Uniqueness: Tin (II) oleate is unique due to its specific coordination chemistry with oleic acid, which imparts distinct properties such as solubility in non-aqueous solvents and specific catalytic activities. Its relatively low toxicity compared to other organotin compounds makes it suitable for medical applications .
Properties
Molecular Formula |
C36H66O4Sn |
---|---|
Molecular Weight |
681.6 g/mol |
IUPAC Name |
bis[[(Z)-octadec-9-enoyl]oxy]tin |
InChI |
InChI=1S/2C18H34O2.Sn/c2*1-2-3-4-5-6-7-8-9-10-11-12-13-14-15-16-17-18(19)20;/h2*9-10H,2-8,11-17H2,1H3,(H,19,20);/q;;+2/p-2/b2*10-9-; |
InChI Key |
PXRFIHSUMBQIOK-CVBJKYQLSA-L |
Isomeric SMILES |
CCCCCCCC/C=C\CCCCCCCC(=O)O[Sn]OC(=O)CCCCCCC/C=C\CCCCCCCC |
Canonical SMILES |
CCCCCCCCC=CCCCCCCCC(=O)O[Sn]OC(=O)CCCCCCCC=CCCCCCCCC |
Origin of Product |
United States |
Disclaimer and Information on In-Vitro Research Products
Please be aware that all articles and product information presented on BenchChem are intended solely for informational purposes. The products available for purchase on BenchChem are specifically designed for in-vitro studies, which are conducted outside of living organisms. In-vitro studies, derived from the Latin term "in glass," involve experiments performed in controlled laboratory settings using cells or tissues. It is important to note that these products are not categorized as medicines or drugs, and they have not received approval from the FDA for the prevention, treatment, or cure of any medical condition, ailment, or disease. We must emphasize that any form of bodily introduction of these products into humans or animals is strictly prohibited by law. It is essential to adhere to these guidelines to ensure compliance with legal and ethical standards in research and experimentation.