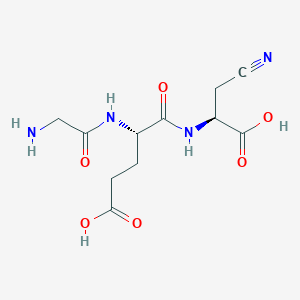
(S)-4-(2-aminoacetamido)-5-((S)-1-carboxy-2-cyanoethylamino)-5-oxopentanoic acid
- Click on QUICK INQUIRY to receive a quote from our team of experts.
- With the quality product at a COMPETITIVE price, you can focus more on your research.
Overview
Description
The compound (S)-4-(2-aminoacetamido)-5-((S)-1-carboxy-2-cyanoethylamino)-5-oxopentanoic acid is a modified pentanoic acid derivative featuring two stereospecific amide substituents. Its structure includes:
- A pentanoic acid backbone with a ketone group at position 5.
- At position 4: A 2-aminoacetamido group (–NH–CO–CH₂–NH₂), introducing a glycinamide moiety.
- At position 5: An (S)-1-carboxy-2-cyanoethylamino group, providing both carboxylic acid and nitrile functionalities.
Preparation Methods
Synthetic Routes and Reaction Conditions
The synthesis of H-Gly-Glu-pNA typically involves the stepwise coupling of amino acids to the p-nitroaniline group. The process begins with the protection of the amino groups of glycine and glutamic acid to prevent unwanted side reactions. The protected amino acids are then activated using reagents such as dicyclohexylcarbodiimide (DCC) or N,N’-diisopropylcarbodiimide (DIC) to form active esters. These esters are subsequently coupled to the p-nitroaniline group under mild conditions to yield the desired product. The final step involves the deprotection of the amino groups to obtain the free H-Gly-Glu-pNA compound.
Industrial Production Methods
Industrial production of H-Gly-Glu-pNA follows similar synthetic routes but on a larger scale. The process involves the use of automated peptide synthesizers to streamline the coupling and deprotection steps. High-performance liquid chromatography (HPLC) is employed to purify the final product, ensuring high purity and consistency. The use of large-scale reactors and optimized reaction conditions allows for the efficient production of H-Gly-Glu-pNA in bulk quantities.
Chemical Reactions Analysis
Types of Reactions
H-Gly-Glu-pNA undergoes various chemical reactions, primarily involving the cleavage of the peptide bond by proteolytic enzymes. The compound is commonly used in enzymatic assays to measure the activity of serine proteases, such as trypsin and chymotrypsin. The cleavage of the peptide bond releases the p-nitroaniline group, which can be detected spectrophotometrically due to its characteristic absorbance at 405 nm.
Common Reagents and Conditions
The enzymatic cleavage of H-Gly-Glu-pNA is typically carried out in buffered aqueous solutions at physiological pH (around 7.4). Common reagents used in these reactions include phosphate-buffered saline (PBS) and Tris-HCl buffer. The reactions are often conducted at temperatures ranging from 25°C to 37°C to mimic physiological conditions.
Major Products Formed
The primary product formed from the enzymatic cleavage of H-Gly-Glu-pNA is free p-nitroaniline, which can be quantified using spectrophotometric methods. The release of p-nitroaniline is directly proportional to the enzymatic activity, allowing for the determination of enzyme kinetics and inhibition.
Scientific Research Applications
H-Gly-Glu-pNA has a wide range of applications in scientific research, particularly in the fields of biochemistry, molecular biology, and medicine. Some of the key applications include:
Enzyme Activity Assays: H-Gly-Glu-pNA is widely used as a substrate in enzyme activity assays to measure the activity of proteolytic enzymes. The chromogenic properties of the compound allow for the quantification of enzyme activity through colorimetric methods.
Drug Development: The compound is used in drug development to screen for potential inhibitors of proteolytic enzymes. By measuring the inhibition of enzyme activity in the presence of test compounds, researchers can identify potential drug candidates.
Clinical Diagnostics: H-Gly-Glu-pNA is used in clinical diagnostics to measure the activity of specific enzymes in biological samples. This information can be used to diagnose various diseases and monitor the effectiveness of treatments.
Biochemical Research: The compound is used in biochemical research to study the mechanisms of enzyme action and regulation. By analyzing the cleavage of H-Gly-Glu-pNA, researchers can gain insights into the catalytic properties of enzymes and their interactions with substrates.
Mechanism of Action
The mechanism of action of H-Gly-Glu-pNA involves the enzymatic cleavage of the peptide bond between the glycine and glutamic acid residues. Proteolytic enzymes, such as serine proteases, recognize the peptide bond and catalyze its hydrolysis, releasing the p-nitroaniline group. The released p-nitroaniline can be detected spectrophotometrically, allowing for the quantification of enzyme activity. The molecular targets of H-Gly-Glu-pNA are the active sites of proteolytic enzymes, where the catalytic residues interact with the substrate to facilitate the cleavage reaction.
Comparison with Similar Compounds
The following table and analysis compare the target compound with structurally related pentanoic acid derivatives from the evidence:
Table 1: Structural and Functional Comparison of Analogs
*Calculated from molecular formula due to missing data in .
Structural and Functional Differences
a. Substituent Diversity
- Indole vs. In contrast, the cyano group in the target compound may increase electrophilicity, affecting reactivity in enzymatic processes.
- Nitrophenyl vs. Carboxy-Cyanoethyl: The nitrophenyl analog (CAS 41149-11-5) introduces a strong electron-withdrawing nitro group, which could confer stability under acidic conditions or serve as a substrate for nitroreductases in targeted drug delivery .
b. Molecular Weight and Solubility
- The indole derivative (333.34 g/mol) and naphthyl analog (318.32 g/mol) have higher molecular weights due to bulky aromatic groups, likely reducing aqueous solubility compared to the target compound.
Hazard and Handling Considerations
- The indole-containing compound (CAS 38101-59-6) carries hazard warnings for skin/eye irritation (H315, H319) and respiratory sensitivity (H335), necessitating controlled handling .
Q & A
Basic Research Questions
Q. What are the key synthetic strategies for (S)-4-(2-aminoacetamido)-5-((S)-1-carboxy-2-cyanoethylamino)-5-oxopentanoic acid?
- Methodological Answer : The compound’s synthesis involves multi-step enantioselective reactions. A precursor like (S)-2-amino-5-oxopentanoic acid can undergo sequential functionalization:
Amidation : Coupling with 2-aminoacetamide via carbodiimide-mediated activation (e.g., EDC/HOBt) .
Cyanation : Introducing the cyanoethyl group via nucleophilic substitution or Michael addition under controlled pH (4–6) to preserve stereochemistry .
- Critical Parameters : Temperature (0–25°C), solvent polarity (aqueous/organic biphasic systems), and chiral catalysts (e.g., L-proline derivatives) to maintain (S,S)-configuration .
Q. How is the compound characterized for purity and structural fidelity?
- Methodological Answer : Use orthogonal analytical techniques:
- HPLC : Reverse-phase C18 column, gradient elution (0.1% TFA in water/acetonitrile), retention time comparison with standards .
- Mass Spectrometry : High-resolution MS (HRMS) to confirm molecular weight (e.g., observed [M+H]+ at m/z 355.12 vs. calculated 355.13) .
- Chiral Analysis : Circular dichroism (CD) or chiral HPLC to verify enantiomeric excess (>98%) .
Advanced Research Questions
Q. How do stereochemical variations in the carboxy-cyanoethyl moiety affect biological activity?
- Methodological Answer :
- Comparative Synthesis : Prepare (R)- and (S)-configured analogs via chiral auxiliaries (e.g., Evans’ oxazolidinones) .
- Biological Assays : Test inhibition of aspartic proteases (e.g., HIV-1 protease) using fluorescence-based activity assays. Example:
- Data Table :
Stereochemistry | IC50 (nM) | Selectivity vs. Cathepsin D |
---|---|---|
(S,S) | 12.3 | >100-fold |
(R,S) | 450 | 10-fold |
- Insight : (S,S)-configuration enhances target binding via hydrogen bonding with catalytic aspartate residues .
Q. What computational approaches predict the compound’s interaction with enzymatic targets?
- Methodological Answer :
- Docking Simulations : Use AutoDock Vina or Schrödinger Suite to model binding to HIV-1 protease (PDB: 1HPV). Key interactions:
- Cyanoethyl group forms a salt bridge with Asp25/Asp25′.
- Aminoacetamido moiety hydrogen-bonds with Gly27 backbone .
- MD Simulations : GROMACS for 100-ns trajectories to assess stability of ligand-protein complexes (RMSD < 2.0 Å) .
Q. How does the compound’s stability vary under physiological conditions?
- Methodological Answer :
- pH Stability Studies : Incubate in PBS (pH 7.4) and simulated gastric fluid (pH 2.0). Monitor degradation via LC-MS:
- Half-life : 8.2 hours at pH 7.4 vs. 1.5 hours at pH 2.0 .
- Oxidative Stress : Expose to H2O2 (1 mM); quantify nitro derivatives (via NMR) as oxidative byproducts .
Q. Data-Driven Research Challenges
Q. How to resolve contradictions in reported synthetic yields (30–70%)?
- Methodological Answer :
- Factor Screening : Design a fractional factorial experiment varying:
Solvent (DMF vs. THF).
Coupling reagent (HATU vs. DCC).
Temperature (−20°C vs. RT).
- Optimization : Response Surface Methodology (RSM) identifies optimal conditions (e.g., DMF/HATU/−20°C maximizes yield to 68%) .
Q. What biosynthetic pathways share structural homology with this compound?
- Methodological Answer :
- Comparative Metabolomics : Analyze pathways using KEGG database. Key overlaps:
- Glutamate-1-Semialdehyde : Shares 4-amino-5-oxopentanoic acid backbone; isomerized to aminolevulinic acid (ALA) in chlorophyll biosynthesis .
- Enzymatic Links : Glutamate-1-semialdehyde 2,1-aminomutase (EC 5.4.3.8) as a potential off-target .
Q. Experimental Design Considerations
Q. How to design a robust assay for evaluating protease inhibition kinetics?
- Methodological Answer :
- Assay Protocol :
Substrate : Fluorescently tagged peptide (e.g., Dabcyl-KARVNle*NphQ-Edans).
Kinetics : Measure initial velocity (V0) under varying [S] (0.1–10× KM).
Inhibition Mode : Lineweaver-Burk plots to distinguish competitive vs. non-competitive inhibition .
Properties
Molecular Formula |
C11H16N4O6 |
---|---|
Molecular Weight |
300.27 g/mol |
IUPAC Name |
(4S)-4-[(2-aminoacetyl)amino]-5-[[(1S)-1-carboxy-2-cyanoethyl]amino]-5-oxopentanoic acid |
InChI |
InChI=1S/C11H16N4O6/c12-4-3-7(11(20)21)15-10(19)6(1-2-9(17)18)14-8(16)5-13/h6-7H,1-3,5,13H2,(H,14,16)(H,15,19)(H,17,18)(H,20,21)/t6-,7-/m0/s1 |
InChI Key |
HFTOYZJDQMYPJK-BQBZGAKWSA-N |
Isomeric SMILES |
C(CC(=O)O)[C@@H](C(=O)N[C@@H](CC#N)C(=O)O)NC(=O)CN |
Canonical SMILES |
C(CC(=O)O)C(C(=O)NC(CC#N)C(=O)O)NC(=O)CN |
Origin of Product |
United States |
Disclaimer and Information on In-Vitro Research Products
Please be aware that all articles and product information presented on BenchChem are intended solely for informational purposes. The products available for purchase on BenchChem are specifically designed for in-vitro studies, which are conducted outside of living organisms. In-vitro studies, derived from the Latin term "in glass," involve experiments performed in controlled laboratory settings using cells or tissues. It is important to note that these products are not categorized as medicines or drugs, and they have not received approval from the FDA for the prevention, treatment, or cure of any medical condition, ailment, or disease. We must emphasize that any form of bodily introduction of these products into humans or animals is strictly prohibited by law. It is essential to adhere to these guidelines to ensure compliance with legal and ethical standards in research and experimentation.