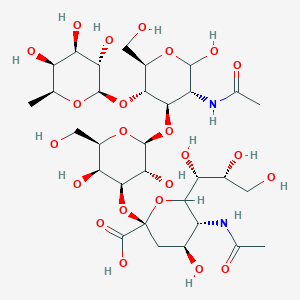
3'-Sialyl-Lewis-a tetrasaccharide
- Click on QUICK INQUIRY to receive a quote from our team of experts.
- With the quality product at a COMPETITIVE price, you can focus more on your research.
Overview
Description
3’-Sialyl-Lewis-a tetrasaccharide, also known as α-NeuNAc-(2→3)-β-D-Gal-(1→3)-(α-L-Fuc-[1→4])-D-GlcNAc, is a complex carbohydrate molecule. It is a tetrasaccharide, meaning it consists of four monosaccharide units. This compound plays a crucial role in cell-to-cell recognition processes and is known to support E-, L-, and P-selectin-dependent adhesion .
Preparation Methods
Synthetic Routes and Reaction Conditions: The synthesis of 3’-Sialyl-Lewis-a tetrasaccharide involves both chemical and chemoenzymatic methods. The chemical synthesis includes the highly stereoselective construction of 1,2-cis-α-L-fucoside and α-D-sialoside, as well as the assembly of the 3,4-disubstituted N-acetylglucosamine subunit. Perbenzylated thiofucoside and N-acetyl-5-N,4-O-oxazolidinone protected sialic acid thioglycoside are employed as glycosyl donors for the efficient preparation of the desired α-fucoside and α-sialoside .
Industrial Production Methods: In industrial settings, a chemoenzymatic approach is often used. The chemically obtained free disaccharide is sequentially sialylated and fucosylated in an enzyme-catalyzed regio- and stereospecific manner to form the target molecule in high yields .
Chemical Reactions Analysis
Types of Reactions: 3’-Sialyl-Lewis-a tetrasaccharide undergoes various chemical reactions, including glycosylation, oxidation, and hydrolysis.
Common Reagents and Conditions:
Glycosylation: Perbenzylated thiofucoside and N-acetyl-5-N,4-O-oxazolidinone protected sialic acid thioglycoside are used as glycosyl donors.
Oxidation: Treatment with LiOH and H2O2 for hydrolysis of methyl ester in the sialic acid.
Hydrolysis: Heating with concentrated ammonia at 50°C to remove acetyl protecting groups.
Major Products: The major products formed from these reactions include the desired α-fucoside and α-sialoside, which are essential components of the tetrasaccharide structure .
Scientific Research Applications
3’-Sialyl-Lewis-a tetrasaccharide has a wide range of applications in scientific research:
Chemistry: Used in the synthesis of complex carbohydrates and glycoconjugates.
Biology: Plays a role in cell-to-cell recognition and adhesion processes.
Medicine: Serves as a tumor marker, particularly in the management of pancreatic cancer.
Industry: Utilized in the development of vaccines and therapeutic agents.
Mechanism of Action
The compound exerts its effects by binding to selectins, which are cell adhesion molecules. This binding supports E-, L-, and P-selectin-dependent adhesion, facilitating cell-to-cell interactions. The molecular targets include endothelial cells and leukocytes, and the pathways involved are primarily related to immune response and inflammation .
Comparison with Similar Compounds
3’-Sialyl-Lewis-a tetrasaccharide is often compared with other similar compounds such as:
3’-Sialyl-Lewis-X tetrasaccharide: Similar in structure but differs in the linkage of the fucose unit.
Sialyl Lewis x: Another tetrasaccharide that plays a role in cell adhesion and immune response.
CA19-9 (Sialyl-Lewis A):
These compounds share similar functions in cell adhesion and recognition but differ in their specific structures and applications.
Properties
Molecular Formula |
C31H52N2O23 |
---|---|
Molecular Weight |
820.7 g/mol |
IUPAC Name |
(2S,4S,5R)-5-acetamido-2-[(2R,3R,4S,5S,6R)-2-[(3R,4R,5S,6R)-3-acetamido-2-hydroxy-6-(hydroxymethyl)-5-[(2R,3S,4R,5S,6S)-3,4,5-trihydroxy-6-methyloxan-2-yl]oxyoxan-4-yl]oxy-3,5-dihydroxy-6-(hydroxymethyl)oxan-4-yl]oxy-4-hydroxy-6-[(1R,2R)-1,2,3-trihydroxypropyl]oxane-2-carboxylic acid |
InChI |
InChI=1S/C31H52N2O23/c1-8-17(41)20(44)21(45)28(50-8)53-23-14(7-36)51-27(47)16(33-10(3)38)25(23)54-29-22(46)26(19(43)13(6-35)52-29)56-31(30(48)49)4-11(39)15(32-9(2)37)24(55-31)18(42)12(40)5-34/h8,11-29,34-36,39-47H,4-7H2,1-3H3,(H,32,37)(H,33,38)(H,48,49)/t8-,11-,12+,13+,14+,15+,16+,17+,18+,19-,20+,21-,22+,23+,24?,25+,26-,27?,28+,29-,31-/m0/s1 |
InChI Key |
XBSNXOHQOTUENA-KBQOLJPHSA-N |
Isomeric SMILES |
C[C@H]1[C@H]([C@H]([C@@H]([C@H](O1)O[C@@H]2[C@H](OC([C@@H]([C@H]2O[C@H]3[C@@H]([C@H]([C@H]([C@H](O3)CO)O)O[C@@]4(C[C@@H]([C@H](C(O4)[C@@H]([C@@H](CO)O)O)NC(=O)C)O)C(=O)O)O)NC(=O)C)O)CO)O)O)O |
Canonical SMILES |
CC1C(C(C(C(O1)OC2C(OC(C(C2OC3C(C(C(C(O3)CO)O)OC4(CC(C(C(O4)C(C(CO)O)O)NC(=O)C)O)C(=O)O)O)NC(=O)C)O)CO)O)O)O |
Origin of Product |
United States |
Disclaimer and Information on In-Vitro Research Products
Please be aware that all articles and product information presented on BenchChem are intended solely for informational purposes. The products available for purchase on BenchChem are specifically designed for in-vitro studies, which are conducted outside of living organisms. In-vitro studies, derived from the Latin term "in glass," involve experiments performed in controlled laboratory settings using cells or tissues. It is important to note that these products are not categorized as medicines or drugs, and they have not received approval from the FDA for the prevention, treatment, or cure of any medical condition, ailment, or disease. We must emphasize that any form of bodily introduction of these products into humans or animals is strictly prohibited by law. It is essential to adhere to these guidelines to ensure compliance with legal and ethical standards in research and experimentation.