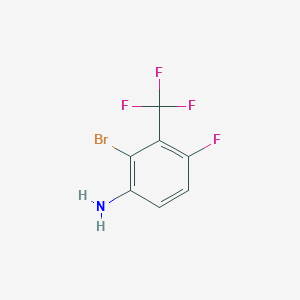
2-Bromo-4-fluoro-3-(trifluoromethyl)aniline
Overview
Description
2-Bromo-4-fluoro-3-(trifluoromethyl)aniline is an aromatic compound characterized by the presence of bromine, fluorine, and trifluoromethyl groups attached to an aniline ring. This compound is of significant interest in organic chemistry due to its unique structural features and reactivity, making it a valuable building block in various chemical syntheses and industrial applications .
Mechanism of Action
Target of Action
Similar compounds have been used in the preparation of monoselective agonists of the sphingosine-1-phosphate receptor . This receptor plays a crucial role in the regulation of immune responses and vascular integrity .
Biochemical Pathways
If the compound acts as an agonist of the sphingosine-1-phosphate receptor, it could potentially influence sphingolipid metabolism and related signaling pathways .
Pharmacokinetics
The compound’s physical properties, such as its boiling point and density , suggest that it could be absorbed and distributed in the body
Result of Action
Similar compounds have been noted to have antimicrobial effects , suggesting that this compound may also exhibit similar properties.
Action Environment
The action, efficacy, and stability of 2-Bromo-4-fluoro-3-(trifluoromethyl)aniline can be influenced by various environmental factors. For instance, the compound should be stored in a cool, dry, and well-ventilated place away from strong oxidizing agents . These conditions can help maintain the compound’s stability and efficacy.
Biochemical Analysis
Biochemical Properties
2-Bromo-4-fluoro-3-(trifluoromethyl)aniline plays a role in biochemical reactions primarily as a substrate or inhibitor. It interacts with various enzymes and proteins, influencing their activity. For instance, it has been used in the preparation of compounds that act as agonists for the sphingosine-1-phosphate receptor . This receptor is involved in numerous cellular processes, including cell growth, survival, and migration. The interactions of this compound with these biomolecules are typically characterized by binding to specific active sites, leading to either activation or inhibition of the enzyme’s function.
Cellular Effects
The effects of this compound on various cell types and cellular processes are diverse. This compound can influence cell function by modulating cell signaling pathways, gene expression, and cellular metabolism. For example, its interaction with the sphingosine-1-phosphate receptor can lead to altered signaling pathways that affect cell proliferation and apoptosis . Additionally, this compound may impact gene expression by binding to transcription factors or other regulatory proteins, thereby influencing the transcription of specific genes involved in cellular metabolism and function.
Molecular Mechanism
At the molecular level, this compound exerts its effects through various mechanisms. It can bind to specific biomolecules, such as enzymes or receptors, altering their activity. For instance, its role as an agonist for the sphingosine-1-phosphate receptor involves binding to the receptor’s active site, leading to receptor activation and subsequent downstream signaling . Additionally, this compound may inhibit certain enzymes by binding to their active sites, preventing substrate access and thus inhibiting enzyme activity. These interactions can result in changes in gene expression, either by directly affecting transcription factors or by modulating signaling pathways that regulate gene transcription.
Temporal Effects in Laboratory Settings
The stability and degradation of this compound in laboratory settings are crucial for its effective use in biochemical studies. Over time, this compound may undergo degradation, leading to a decrease in its efficacy. Studies have shown that it remains stable under specific storage conditions, such as in a dark place and at room temperature . Prolonged exposure to light or extreme temperatures can result in its degradation, affecting its long-term effects on cellular function. In vitro and in vivo studies have demonstrated that the compound’s activity can diminish over time, necessitating careful handling and storage to maintain its stability.
Dosage Effects in Animal Models
The effects of this compound vary with different dosages in animal models. At lower doses, it may exhibit minimal toxicity and exert its intended biochemical effects, such as receptor activation or enzyme inhibition . At higher doses, the compound can cause toxic or adverse effects, including cellular damage and disruption of normal cellular processes. Threshold effects have been observed, where a specific dosage range is required to achieve the desired biochemical activity without inducing toxicity. These findings highlight the importance of dosage optimization in experimental studies involving this compound.
Metabolic Pathways
This compound is involved in various metabolic pathways, interacting with enzymes and cofactors that facilitate its metabolism. The compound undergoes biotransformation, leading to the formation of metabolites that can be excreted from the body . Enzymes such as cytochrome P450 play a crucial role in its metabolism, catalyzing reactions that modify the compound’s structure and enhance its solubility for excretion. These metabolic pathways can influence the compound’s efficacy and toxicity, as the metabolites may have different biochemical properties compared to the parent compound.
Transport and Distribution
The transport and distribution of this compound within cells and tissues are mediated by specific transporters and binding proteins. Once inside the cell, the compound can interact with intracellular proteins that facilitate its movement to specific cellular compartments . This distribution is essential for its biochemical activity, as it needs to reach its target sites, such as receptors or enzymes, to exert its effects. The compound’s localization and accumulation within tissues can also impact its overall efficacy and potential toxicity.
Subcellular Localization
The subcellular localization of this compound is determined by targeting signals and post-translational modifications that direct it to specific compartments or organelles. For instance, the compound may be directed to the endoplasmic reticulum, mitochondria, or nucleus, depending on its biochemical role . This localization is crucial for its activity, as it needs to be in proximity to its target biomolecules to exert its effects. Additionally, post-translational modifications, such as phosphorylation or ubiquitination, can influence the compound’s localization and stability within the cell.
Preparation Methods
Synthetic Routes and Reaction Conditions: The synthesis of 2-Bromo-4-fluoro-3-(trifluoromethyl)aniline typically involves multi-step reactions starting from commercially available precursors. One common method includes the bromination of 4-fluoro-3-(trifluoromethyl)aniline using N-bromosuccinimide (NBS) in the presence of a solvent like dichloromethane .
Industrial Production Methods: Industrial production of this compound may involve optimized reaction conditions to ensure high yield and purity. This includes controlling the temperature, reaction time, and the use of catalysts to facilitate the bromination process efficiently .
Chemical Reactions Analysis
Types of Reactions: 2-Bromo-4-fluoro-3-(trifluoromethyl)aniline undergoes various chemical reactions, including:
Substitution Reactions: The bromine atom can be replaced by other nucleophiles under suitable conditions.
Oxidation and Reduction: The compound can participate in oxidation and reduction reactions, altering the oxidation state of the functional groups.
Common Reagents and Conditions:
Nucleophilic Substitution: Reagents like sodium methoxide or potassium tert-butoxide in polar aprotic solvents.
Oxidation: Reagents such as potassium permanganate or chromium trioxide.
Reduction: Catalysts like palladium on carbon (Pd/C) in the presence of hydrogen gas.
Major Products: The products formed depend on the specific reaction conditions and reagents used. For instance, nucleophilic substitution can yield various substituted anilines, while oxidation and reduction can lead to different functionalized derivatives .
Scientific Research Applications
2-Bromo-4-fluoro-3-(trifluoromethyl)aniline has diverse applications in scientific research:
Chemistry: Used as a building block in the synthesis of complex organic molecules.
Biology: Investigated for its potential biological activities and interactions with biomolecules.
Industry: Utilized in the production of agrochemicals, dyes, and advanced materials.
Comparison with Similar Compounds
- 4-Bromo-3-(trifluoromethyl)aniline
- 2-Bromo-5-(trifluoromethyl)aniline
- 4-Fluoro-3-(trifluoromethyl)aniline
Comparison: Compared to its analogs, 2-Bromo-4-fluoro-3-(trifluoromethyl)aniline is unique due to the specific positioning of the bromine and fluorine atoms, which can significantly influence its reactivity and interaction with other molecules. This distinct arrangement makes it particularly valuable in certain synthetic applications and research contexts .
Properties
IUPAC Name |
2-bromo-4-fluoro-3-(trifluoromethyl)aniline | |
---|---|---|
Source | PubChem | |
URL | https://pubchem.ncbi.nlm.nih.gov | |
Description | Data deposited in or computed by PubChem | |
InChI |
InChI=1S/C7H4BrF4N/c8-6-4(13)2-1-3(9)5(6)7(10,11)12/h1-2H,13H2 | |
Source | PubChem | |
URL | https://pubchem.ncbi.nlm.nih.gov | |
Description | Data deposited in or computed by PubChem | |
InChI Key |
JTPACHMIKOCFFN-UHFFFAOYSA-N | |
Source | PubChem | |
URL | https://pubchem.ncbi.nlm.nih.gov | |
Description | Data deposited in or computed by PubChem | |
Canonical SMILES |
C1=CC(=C(C(=C1N)Br)C(F)(F)F)F | |
Source | PubChem | |
URL | https://pubchem.ncbi.nlm.nih.gov | |
Description | Data deposited in or computed by PubChem | |
Molecular Formula |
C7H4BrF4N | |
Source | PubChem | |
URL | https://pubchem.ncbi.nlm.nih.gov | |
Description | Data deposited in or computed by PubChem | |
Molecular Weight |
258.01 g/mol | |
Source | PubChem | |
URL | https://pubchem.ncbi.nlm.nih.gov | |
Description | Data deposited in or computed by PubChem | |
Retrosynthesis Analysis
AI-Powered Synthesis Planning: Our tool employs the Template_relevance Pistachio, Template_relevance Bkms_metabolic, Template_relevance Pistachio_ringbreaker, Template_relevance Reaxys, Template_relevance Reaxys_biocatalysis model, leveraging a vast database of chemical reactions to predict feasible synthetic routes.
One-Step Synthesis Focus: Specifically designed for one-step synthesis, it provides concise and direct routes for your target compounds, streamlining the synthesis process.
Accurate Predictions: Utilizing the extensive PISTACHIO, BKMS_METABOLIC, PISTACHIO_RINGBREAKER, REAXYS, REAXYS_BIOCATALYSIS database, our tool offers high-accuracy predictions, reflecting the latest in chemical research and data.
Strategy Settings
Precursor scoring | Relevance Heuristic |
---|---|
Min. plausibility | 0.01 |
Model | Template_relevance |
Template Set | Pistachio/Bkms_metabolic/Pistachio_ringbreaker/Reaxys/Reaxys_biocatalysis |
Top-N result to add to graph | 6 |
Feasible Synthetic Routes
Disclaimer and Information on In-Vitro Research Products
Please be aware that all articles and product information presented on BenchChem are intended solely for informational purposes. The products available for purchase on BenchChem are specifically designed for in-vitro studies, which are conducted outside of living organisms. In-vitro studies, derived from the Latin term "in glass," involve experiments performed in controlled laboratory settings using cells or tissues. It is important to note that these products are not categorized as medicines or drugs, and they have not received approval from the FDA for the prevention, treatment, or cure of any medical condition, ailment, or disease. We must emphasize that any form of bodily introduction of these products into humans or animals is strictly prohibited by law. It is essential to adhere to these guidelines to ensure compliance with legal and ethical standards in research and experimentation.