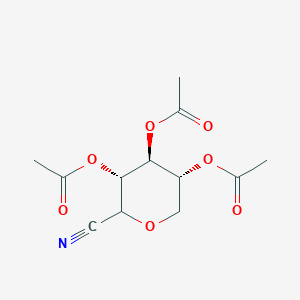
Tri-O-acetyl-D-xylopyranosyl cyanide
- Click on QUICK INQUIRY to receive a quote from our team of experts.
- With the quality product at a COMPETITIVE price, you can focus more on your research.
Overview
Description
Tri-O-acetyl-D-xylopyranosyl cyanide is a chemical compound with the molecular formula C12H15NO7 It is a derivative of D-xylopyranose, where three hydroxyl groups are acetylated, and one hydroxyl group is replaced by a cyanide group
Preparation Methods
Synthetic Routes and Reaction Conditions
Tri-O-acetyl-D-xylopyranosyl cyanide can be synthesized through the acetylation of D-xylopyranose followed by the introduction of a cyanide group. The acetylation process typically involves the reaction of D-xylopyranose with acetic anhydride in the presence of a catalyst such as pyridine. The reaction conditions usually include a temperature range of 0-25°C and a reaction time of several hours.
The introduction of the cyanide group can be achieved through the reaction of the acetylated D-xylopyranose with a cyanide source such as sodium cyanide or potassium cyanide. This reaction is typically carried out in an organic solvent such as dimethyl sulfoxide (DMSO) or acetonitrile, under mild conditions.
Industrial Production Methods
Industrial production of this compound follows similar synthetic routes but on a larger scale. The process involves the use of industrial-grade reagents and optimized reaction conditions to ensure high yield and purity of the final product. The use of continuous flow reactors and automated systems can enhance the efficiency and scalability of the production process.
Chemical Reactions Analysis
Types of Reactions
Tri-O-acetyl-D-xylopyranosyl cyanide undergoes various types of chemical reactions, including:
Oxidation: The compound can be oxidized to form corresponding carboxylic acids or other oxidized derivatives.
Reduction: Reduction reactions can convert the cyanide group to an amine or other reduced forms.
Substitution: The acetyl groups can be substituted with other functional groups through nucleophilic substitution reactions.
Common Reagents and Conditions
Oxidation: Common oxidizing agents include potassium permanganate (KMnO4) and chromium trioxide (CrO3). The reactions are typically carried out in aqueous or organic solvents under controlled temperatures.
Reduction: Reducing agents such as lithium aluminum hydride (LiAlH4) or sodium borohydride (NaBH4) are used in organic solvents like ether or tetrahydrofuran (THF).
Substitution: Nucleophiles such as hydroxide ions (OH-) or amines (NH2-) can be used to replace the acetyl groups. These reactions are often conducted in polar solvents like water or alcohols.
Major Products Formed
Oxidation: Carboxylic acids, aldehydes, or ketones.
Reduction: Amines or other reduced derivatives.
Substitution: Hydroxylated or aminated derivatives.
Scientific Research Applications
Tri-O-acetyl-D-xylopyranosyl cyanide has several scientific research applications, including:
Chemistry: It is used as a building block in the synthesis of more complex molecules and as a reagent in organic synthesis.
Biology: The compound is studied for its potential biological activities and interactions with biomolecules.
Medicine: Research is ongoing to explore its potential therapeutic applications, including its use as a precursor for drug development.
Industry: It is used in the production of specialty chemicals and as an intermediate in various industrial processes.
Mechanism of Action
The mechanism of action of Tri-O-acetyl-D-xylopyranosyl cyanide involves its interaction with specific molecular targets and pathways. The acetyl groups and cyanide moiety can participate in various biochemical reactions, influencing cellular processes and enzyme activities. The exact molecular targets and pathways depend on the specific application and context of use.
Comparison with Similar Compounds
Similar Compounds
- Tri-O-acetyl-D-glucopyranosyl cyanide
- Tri-O-acetyl-D-galactopyranosyl cyanide
- Tri-O-acetyl-D-mannopyranosyl cyanide
Uniqueness
Tri-O-acetyl-D-xylopyranosyl cyanide is unique due to its specific structural features, including the arrangement of acetyl groups and the presence of a cyanide moiety. This unique structure imparts distinct chemical properties and reactivity, making it valuable for specific applications in research and industry.
Properties
Molecular Formula |
C12H15NO7 |
---|---|
Molecular Weight |
285.25 g/mol |
IUPAC Name |
[(3R,4S,5S)-4,5-diacetyloxy-6-cyanooxan-3-yl] acetate |
InChI |
InChI=1S/C12H15NO7/c1-6(14)18-10-5-17-9(4-13)11(19-7(2)15)12(10)20-8(3)16/h9-12H,5H2,1-3H3/t9?,10-,11+,12+/m1/s1 |
InChI Key |
YJBGGXBOXBPJPU-WSWARRKISA-N |
Isomeric SMILES |
CC(=O)O[C@@H]1COC([C@@H]([C@H]1OC(=O)C)OC(=O)C)C#N |
Canonical SMILES |
CC(=O)OC1COC(C(C1OC(=O)C)OC(=O)C)C#N |
Origin of Product |
United States |
Disclaimer and Information on In-Vitro Research Products
Please be aware that all articles and product information presented on BenchChem are intended solely for informational purposes. The products available for purchase on BenchChem are specifically designed for in-vitro studies, which are conducted outside of living organisms. In-vitro studies, derived from the Latin term "in glass," involve experiments performed in controlled laboratory settings using cells or tissues. It is important to note that these products are not categorized as medicines or drugs, and they have not received approval from the FDA for the prevention, treatment, or cure of any medical condition, ailment, or disease. We must emphasize that any form of bodily introduction of these products into humans or animals is strictly prohibited by law. It is essential to adhere to these guidelines to ensure compliance with legal and ethical standards in research and experimentation.