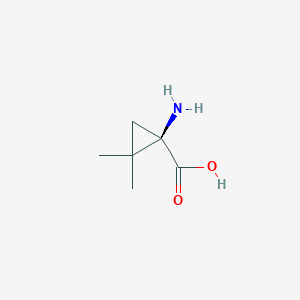
(R)-1-Amino-2,2-dimethylcyclopropanecarboxylic acid
- Click on QUICK INQUIRY to receive a quote from our team of experts.
- With the quality product at a COMPETITIVE price, you can focus more on your research.
Overview
Description
®-1-Amino-2,2-dimethylcyclopropanecarboxylic acid is a chiral amino acid derivative characterized by a cyclopropane ring substituted with an amino group and a carboxylic acid group
Preparation Methods
Synthetic Routes and Reaction Conditions: The synthesis of ®-1-Amino-2,2-dimethylcyclopropanecarboxylic acid can be achieved through several methods:
Alkylation of Glycine Equivalents: This method involves the alkylation of glycine equivalents with 1,2-electrophiles.
Intramolecular Cyclization: Another approach is the intramolecular cyclization of γ-substituted amino acid derivatives.
Cyclopropanation Reactions: Cyclopropanation of alkenes using diazo compounds, ylides, or carbene intermediates is also a viable method.
Industrial Production Methods: Industrial production of this compound typically involves large-scale cyclopropanation reactions, utilizing diazo compounds or ylides under controlled conditions to ensure high yield and purity .
Types of Reactions:
Oxidation: The compound can undergo oxidation reactions, typically using oxidizing agents like potassium permanganate or hydrogen peroxide.
Reduction: Reduction reactions can be performed using reducing agents such as lithium aluminum hydride or sodium borohydride.
Substitution: Nucleophilic substitution reactions can occur, where the amino group can be replaced by other nucleophiles under appropriate conditions.
Common Reagents and Conditions:
Oxidation: Potassium permanganate, hydrogen peroxide.
Reduction: Lithium aluminum hydride, sodium borohydride.
Substitution: Various nucleophiles depending on the desired product.
Major Products:
Oxidation Products: Carboxylic acids or ketones.
Reduction Products: Alcohols or amines.
Substitution Products: Depending on the nucleophile used, a variety of substituted cyclopropane derivatives can be obtained.
Chemistry:
Synthesis of Peptidomimetics: The compound is used as a building block in the synthesis of peptidomimetics, which are molecules that mimic the structure of peptides.
Biology:
Plant Growth Regulators: Derivatives of this compound have been studied for their role as plant growth regulators.
Medicine:
Antiviral Agents:
Industry:
Mechanism of Action
The mechanism of action of ®-1-Amino-2,2-dimethylcyclopropanecarboxylic acid involves its interaction with specific molecular targets, such as enzymes or receptors. The compound can act as an inhibitor or activator of these targets, modulating various biochemical pathways. For example, in antiviral applications, it may inhibit viral proteases, thereby preventing viral replication .
Comparison with Similar Compounds
1-Aminocyclopropanecarboxylic Acid: A non-substituted analog with similar structural features but different reactivity and applications.
Coronamic Acid: Another cyclopropane-containing amino acid with distinct biological activities.
Uniqueness: ®-1-Amino-2,2-dimethylcyclopropanecarboxylic acid is unique due to its chiral nature and the presence of the dimethyl-substituted cyclopropane ring, which imparts specific steric and electronic properties that influence its reactivity and interactions with biological targets .
Properties
Molecular Formula |
C6H11NO2 |
---|---|
Molecular Weight |
129.16 g/mol |
IUPAC Name |
(1R)-1-amino-2,2-dimethylcyclopropane-1-carboxylic acid |
InChI |
InChI=1S/C6H11NO2/c1-5(2)3-6(5,7)4(8)9/h3,7H2,1-2H3,(H,8,9)/t6-/m0/s1 |
InChI Key |
CUDYUNNRMLWYTR-LURJTMIESA-N |
Isomeric SMILES |
CC1(C[C@@]1(C(=O)O)N)C |
Canonical SMILES |
CC1(CC1(C(=O)O)N)C |
Origin of Product |
United States |
Disclaimer and Information on In-Vitro Research Products
Please be aware that all articles and product information presented on BenchChem are intended solely for informational purposes. The products available for purchase on BenchChem are specifically designed for in-vitro studies, which are conducted outside of living organisms. In-vitro studies, derived from the Latin term "in glass," involve experiments performed in controlled laboratory settings using cells or tissues. It is important to note that these products are not categorized as medicines or drugs, and they have not received approval from the FDA for the prevention, treatment, or cure of any medical condition, ailment, or disease. We must emphasize that any form of bodily introduction of these products into humans or animals is strictly prohibited by law. It is essential to adhere to these guidelines to ensure compliance with legal and ethical standards in research and experimentation.