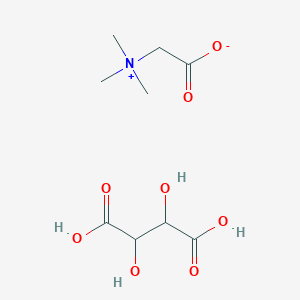
2,3-Dihydroxybutanedioic acid;2-(trimethylazaniumyl)acetate
- Click on QUICK INQUIRY to receive a quote from our team of experts.
- With the quality product at a COMPETITIVE price, you can focus more on your research.
Overview
Description
2,3-Dihydroxybutanedioic acid;2-(trimethylazaniumyl)acetate: is a compound that combines two distinct chemical entities: 2,3-dihydroxybutanedioic acid and 2-(trimethylazaniumyl)acetate. The former is a tetraric acid, commonly known as tartaric acid, which is widely used in the food and pharmaceutical industries. The latter, 2-(trimethylazaniumyl)acetate, is a derivative of betaine, a compound involved in various metabolic processes in living organisms.
Preparation Methods
Synthetic Routes and Reaction Conditions:
2,3-Dihydroxybutanedioic acid: can be synthesized through the fermentation of glucose by certain fungi or bacteria. It can also be obtained by the chemical oxidation of maleic acid or fumaric acid using oxidizing agents like potassium permanganate.
2-(Trimethylazaniumyl)acetate: is typically synthesized by the methylation of glycine using methyl iodide in the presence of a base such as sodium hydroxide.
Industrial Production Methods:
2,3-Dihydroxybutanedioic acid: is industrially produced through the fermentation of carbohydrates by Aspergillus niger. The fermentation broth is then purified to obtain the acid.
2-(Trimethylazaniumyl)acetate: is produced on an industrial scale by the reaction of glycine with trimethylamine, followed by purification processes to isolate the desired product.
Chemical Reactions Analysis
Types of Reactions:
Oxidation: 2,3-Dihydroxybutanedioic acid can undergo oxidation to form oxalic acid and carbon dioxide.
Reduction: It can be reduced to form dihydroxybutanedioic acid derivatives.
Substitution: The hydroxyl groups in 2,3-dihydroxybutanedioic acid can be substituted with other functional groups using appropriate reagents.
Common Reagents and Conditions:
Oxidizing Agents: Potassium permanganate, hydrogen peroxide.
Reducing Agents: Sodium borohydride, lithium aluminum hydride.
Substitution Reagents: Acyl chlorides, alkyl halides.
Major Products:
Oxidation Products: Oxalic acid, carbon dioxide.
Reduction Products: Dihydroxybutanedioic acid derivatives.
Substitution Products: Various esters and ethers of dihydroxybutanedioic acid.
Scientific Research Applications
Chemistry:
- Used as a chiral resolving agent in the separation of enantiomers.
- Employed in the synthesis of various organic compounds.
Biology:
- Acts as a chelating agent in biochemical studies.
- Used in the study of metabolic pathways involving betaine.
Medicine:
- Utilized in the formulation of pharmaceuticals for its antioxidant properties.
- Investigated for its potential role in reducing homocysteine levels in the blood.
Industry:
- Widely used in the food industry as an acidulant and stabilizer.
- Employed in the production of effervescent tablets and other pharmaceutical formulations.
Mechanism of Action
2,3-Dihydroxybutanedioic acid:
- Functions as a chelating agent, binding to metal ions and preventing their participation in unwanted chemical reactions.
- Acts as an antioxidant, neutralizing free radicals and preventing oxidative damage.
2-(Trimethylazaniumyl)acetate:
- Functions as a methyl group donor in various biochemical reactions, including the methylation of homocysteine to form methionine.
- Involved in osmoregulation and the maintenance of cellular hydration.
Comparison with Similar Compounds
Tartaric Acid: Similar to 2,3-dihydroxybutanedioic acid but lacks the additional functional group present in the latter.
Betaine: Similar to 2-(trimethylazaniumyl)acetate but differs in its specific chemical structure and functional groups.
Uniqueness:
- The combination of 2,3-dihydroxybutanedioic acid and 2-(trimethylazaniumyl)acetate in a single compound provides unique properties, such as enhanced chelating ability and improved stability in various applications.
Properties
Molecular Formula |
C9H17NO8 |
---|---|
Molecular Weight |
267.23 g/mol |
IUPAC Name |
2,3-dihydroxybutanedioic acid;2-(trimethylazaniumyl)acetate |
InChI |
InChI=1S/C5H11NO2.C4H6O6/c1-6(2,3)4-5(7)8;5-1(3(7)8)2(6)4(9)10/h4H2,1-3H3;1-2,5-6H,(H,7,8)(H,9,10) |
InChI Key |
LKAOQCIAIYQGFG-UHFFFAOYSA-N |
Canonical SMILES |
C[N+](C)(C)CC(=O)[O-].C(C(C(=O)O)O)(C(=O)O)O |
Origin of Product |
United States |
Disclaimer and Information on In-Vitro Research Products
Please be aware that all articles and product information presented on BenchChem are intended solely for informational purposes. The products available for purchase on BenchChem are specifically designed for in-vitro studies, which are conducted outside of living organisms. In-vitro studies, derived from the Latin term "in glass," involve experiments performed in controlled laboratory settings using cells or tissues. It is important to note that these products are not categorized as medicines or drugs, and they have not received approval from the FDA for the prevention, treatment, or cure of any medical condition, ailment, or disease. We must emphasize that any form of bodily introduction of these products into humans or animals is strictly prohibited by law. It is essential to adhere to these guidelines to ensure compliance with legal and ethical standards in research and experimentation.