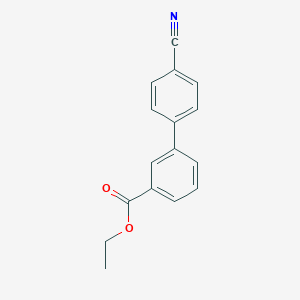
Ethyl 4'-cyanobiphenyl-3-carboxylate
- Click on QUICK INQUIRY to receive a quote from our team of experts.
- With the quality product at a COMPETITIVE price, you can focus more on your research.
Overview
Description
Ethyl 4’-cyanobiphenyl-3-carboxylate is an organic compound with the molecular formula C16H13NO2. It is a derivative of biphenyl, featuring a cyano group at the 4’ position and an ethyl ester group at the 3 position of the biphenyl ring.
Mechanism of Action
Mode of Action
It’s speculated that it might undergo Knoevenagel condensation in certain reactions
Biochemical Pathways
It’s known that the compound can participate in multi-component reactions, potentially affecting various biochemical pathways . More research is needed to fully understand the downstream effects of these interactions.
Preparation Methods
Synthetic Routes and Reaction Conditions: The synthesis of ethyl 4’-cyanobiphenyl-3-carboxylate typically involves the following steps:
Formation of Biphenyl Core: The biphenyl core can be synthesized through a Suzuki coupling reaction between a halogenated benzene and a boronic acid derivative.
Introduction of Cyano Group: The cyano group can be introduced via a nucleophilic substitution reaction using a suitable cyanating agent such as copper(I) cyanide.
Esterification: The carboxyl group is esterified using ethanol in the presence of an acid catalyst to form the ethyl ester.
Industrial Production Methods: Industrial production of ethyl 4’-cyanobiphenyl-3-carboxylate may involve similar synthetic routes but optimized for large-scale production. This includes the use of continuous flow reactors and automated systems to ensure high yield and purity .
Types of Reactions:
Oxidation: Ethyl 4’-cyanobiphenyl-3-carboxylate can undergo oxidation reactions to form corresponding carboxylic acids or other oxidized derivatives.
Reduction: The cyano group can be reduced to an amine group using reducing agents such as lithium aluminum hydride.
Common Reagents and Conditions:
Oxidation: Potassium permanganate or chromium trioxide in acidic conditions.
Reduction: Lithium aluminum hydride or hydrogenation with a palladium catalyst.
Substitution: Halogenating agents like bromine or chlorinating agents in the presence of a Lewis acid catalyst.
Major Products:
Oxidation: Formation of carboxylic acids.
Reduction: Formation of amines.
Substitution: Formation of halogenated biphenyl derivatives.
Scientific Research Applications
Ethyl 4’-cyanobiphenyl-3-carboxylate has several scientific research applications:
Materials Science: Used as a precursor in the synthesis of liquid crystals and other advanced materials.
Pharmaceuticals: Investigated for its potential as an intermediate in the synthesis of biologically active compounds.
Organic Synthesis: Utilized as a building block in the synthesis of complex organic molecules for research purposes.
Comparison with Similar Compounds
Ethyl 4’-bromobiphenyl-3-carboxylate: Similar structure but with a bromine atom instead of a cyano group.
Ethyl 4’-methoxybiphenyl-3-carboxylate: Contains a methoxy group instead of a cyano group.
Uniqueness: Ethyl 4’-cyanobiphenyl-3-carboxylate is unique due to the presence of the cyano group, which imparts distinct electronic properties and reactivity compared to its analogs. This makes it particularly useful in applications requiring specific electronic characteristics .
Properties
IUPAC Name |
ethyl 3-(4-cyanophenyl)benzoate |
Source
|
---|---|---|
Source | PubChem | |
URL | https://pubchem.ncbi.nlm.nih.gov | |
Description | Data deposited in or computed by PubChem | |
InChI |
InChI=1S/C16H13NO2/c1-2-19-16(18)15-5-3-4-14(10-15)13-8-6-12(11-17)7-9-13/h3-10H,2H2,1H3 |
Source
|
Source | PubChem | |
URL | https://pubchem.ncbi.nlm.nih.gov | |
Description | Data deposited in or computed by PubChem | |
InChI Key |
KPLMOZNBQVTKCS-UHFFFAOYSA-N |
Source
|
Source | PubChem | |
URL | https://pubchem.ncbi.nlm.nih.gov | |
Description | Data deposited in or computed by PubChem | |
Canonical SMILES |
CCOC(=O)C1=CC=CC(=C1)C2=CC=C(C=C2)C#N |
Source
|
Source | PubChem | |
URL | https://pubchem.ncbi.nlm.nih.gov | |
Description | Data deposited in or computed by PubChem | |
Molecular Formula |
C16H13NO2 |
Source
|
Source | PubChem | |
URL | https://pubchem.ncbi.nlm.nih.gov | |
Description | Data deposited in or computed by PubChem | |
DSSTOX Substance ID |
DTXSID10618690 |
Source
|
Record name | Ethyl 4'-cyano[1,1'-biphenyl]-3-carboxylate | |
Source | EPA DSSTox | |
URL | https://comptox.epa.gov/dashboard/DTXSID10618690 | |
Description | DSSTox provides a high quality public chemistry resource for supporting improved predictive toxicology. | |
Molecular Weight |
251.28 g/mol |
Source
|
Source | PubChem | |
URL | https://pubchem.ncbi.nlm.nih.gov | |
Description | Data deposited in or computed by PubChem | |
CAS No. |
131379-34-5 |
Source
|
Record name | Ethyl 4'-cyano[1,1'-biphenyl]-3-carboxylate | |
Source | EPA DSSTox | |
URL | https://comptox.epa.gov/dashboard/DTXSID10618690 | |
Description | DSSTox provides a high quality public chemistry resource for supporting improved predictive toxicology. | |
Disclaimer and Information on In-Vitro Research Products
Please be aware that all articles and product information presented on BenchChem are intended solely for informational purposes. The products available for purchase on BenchChem are specifically designed for in-vitro studies, which are conducted outside of living organisms. In-vitro studies, derived from the Latin term "in glass," involve experiments performed in controlled laboratory settings using cells or tissues. It is important to note that these products are not categorized as medicines or drugs, and they have not received approval from the FDA for the prevention, treatment, or cure of any medical condition, ailment, or disease. We must emphasize that any form of bodily introduction of these products into humans or animals is strictly prohibited by law. It is essential to adhere to these guidelines to ensure compliance with legal and ethical standards in research and experimentation.