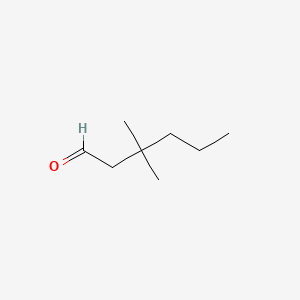
3,3-Dimethylhexanal
Overview
Description
3,3-Dimethylhexanal is an organic compound with the molecular formula C8H16O. It is a colorless liquid with a distinctive aromatic odor. This compound belongs to the family of aldehydes, characterized by the presence of a carbonyl group (C=O) bonded to a hydrogen atom and an alkyl group. It is commonly used as an intermediate in the synthesis of various chemicals and as a fragrance component in perfumes and flavorings .
Preparation Methods
Synthetic Routes and Reaction Conditions
3,3-Dimethylhexanal can be synthesized through the reaction of hexanal with methyl ethyl ketone under acidic conditions. The reaction involves the formation of a carbon-carbon bond between the aldehyde and the ketone, followed by dehydration to yield the desired product .
Industrial Production Methods
In industrial settings, this compound is produced using similar synthetic routes but on a larger scale. The reaction conditions are optimized to ensure high yield and purity of the product. The process typically involves the use of catalysts and controlled temperature and pressure conditions to facilitate the reaction .
Chemical Reactions Analysis
Types of Reactions
3,3-Dimethylhexanal undergoes various chemical reactions, including:
Oxidation: It can be oxidized to form the corresponding carboxylic acid.
Reduction: It can be reduced to form the corresponding alcohol.
Substitution: It can undergo nucleophilic substitution reactions, where the aldehyde group is replaced by other functional groups
Common Reagents and Conditions
Oxidation: Common oxidizing agents include potassium permanganate (KMnO4) and chromium trioxide (CrO3).
Reduction: Common reducing agents include sodium borohydride (NaBH4) and lithium aluminum hydride (LiAlH4).
Substitution: Common reagents include Grignard reagents (RMgX) and organolithium reagents (RLi)
Major Products Formed
Oxidation: 3,3-Dimethylhexanoic acid.
Reduction: 3,3-Dimethylhexanol.
Substitution: Various substituted derivatives depending on the nucleophile used
Scientific Research Applications
Environmental Chemistry
3,3-Dimethylhexanal plays a significant role in atmospheric chemistry. Studies have shown that it can undergo degradation in the presence of atmospheric oxidants such as hydroxyl radicals and chlorine atoms. The degradation products include short-chain carbonyl compounds like acetone and formaldehyde, which are relevant in understanding air quality and photochemical smog formation .
Table 1: Kinetic Parameters of this compound Degradation
Reaction | Rate Coefficient (cm³ molecule⁻¹ s⁻¹) |
---|---|
Cl + this compound | |
OH + this compound |
This degradation pathway indicates that while this compound itself may not significantly impact radiative forcing, its transformation products can contribute to secondary organic aerosol formation, affecting air quality .
Phytochemical Applications
Research indicates that this compound can be utilized in the production of phytochemical compounds that are effective in removing heavy metals from the environment. When cyanobacteria are exposed to aliphatic compounds, they produce phytochemicals capable of chelating heavy metals . This property suggests potential applications in bioremediation strategies.
Flavor and Fragrance Industry
Due to its presence in various herbs and spices, including cinnamon and ginseng, this compound may have applications in the flavor and fragrance industry. Its extraction from natural sources using supercritical carbon dioxide has been noted, where it constitutes a significant percentage of the extracted material .
Toxicological Studies
While this compound is found in natural products, it is also recognized for its potential toxicity. Studies have detected this compound in various biological samples but have not quantified its effects extensively. Understanding its toxicological profile could lead to insights into safe handling practices and regulatory measures .
Industrial Applications
In industrial chemistry, derivatives of compounds related to this compound have been explored for their roles in synthesizing agrochemicals such as fungicides and herbicides. The structural similarities with other aliphatic aldehydes suggest potential pathways for synthesis and application in agricultural chemistry .
Case Study 1: Atmospheric Reactivity
A detailed kinetic study was conducted on the degradation of various carbonyl compounds including this compound under controlled conditions. The results indicated rapid degradation rates upon exposure to atmospheric oxidants, highlighting the compound's role in tropospheric chemistry and its potential environmental implications .
Case Study 2: Phytoremediation Potential
Research into the phytochemical properties of this compound has shown promise for its use in bioremediation efforts aimed at heavy metal removal from contaminated water bodies. Experiments demonstrated that cyanobacteria treated with this compound exhibited increased metal uptake capabilities .
Mechanism of Action
The mechanism of action of 3,3-Dimethylhexanal involves its interaction with various molecular targets and pathways. As an aldehyde, it can form Schiff bases with primary amines, leading to the formation of imines. This reaction is important in biological systems, where it can modify proteins and other biomolecules. Additionally, its carbonyl group can participate in nucleophilic addition reactions, making it a versatile intermediate in organic synthesis .
Comparison with Similar Compounds
Similar Compounds
Hexanal: A straight-chain aldehyde with similar reactivity but lacking the dimethyl substitution.
3,3-Dimethylhexane: An alkane with similar molecular weight but different functional groups and reactivity.
3,3-Dimethylpentanal: A related aldehyde with a shorter carbon chain .
Uniqueness
3,3-Dimethylhexanal is unique due to its specific structure, which includes two methyl groups at the third carbon position. This structural feature influences its reactivity and physical properties, making it distinct from other aldehydes and related compounds .
Biological Activity
3,3-Dimethylhexanal (C8H16O), a branched-chain aldehyde, has garnered attention in the field of chemical biology due to its potential biological activities. This article explores its properties, biological effects, and relevant research findings.
This compound is characterized by its molecular structure which includes two methyl groups attached to the third carbon of a hexanal chain. This structural configuration influences its chemical reactivity and biological interactions. The compound is primarily used in the synthesis of various organic compounds and as a flavoring agent in the food industry.
1. Antioxidant Activity
Research has indicated that this compound exhibits significant antioxidant properties. Antioxidants are crucial for neutralizing free radicals, thus preventing oxidative stress which can lead to cellular damage and various diseases.
- Study Findings : A study evaluating the antioxidant potential of various aldehydes found that this compound demonstrated moderate scavenging activity against free radicals using assays such as DPPH and ABTS .
2. Antimicrobial Activity
The antimicrobial efficacy of this compound has been investigated against various pathogens. Its ability to inhibit microbial growth suggests potential applications in food preservation and pharmaceuticals.
- Research Insights : In vitro studies have shown that this compound possesses antibacterial properties against both Gram-positive and Gram-negative bacteria. The compound's mode of action is believed to disrupt microbial cell membranes, leading to cell lysis .
3. Cytotoxicity
The cytotoxic effects of this compound have been explored in several cancer cell lines. Understanding its potential as an anticancer agent is vital for developing new therapeutic strategies.
- Cytotoxic Studies : Evaluations using MTT assays on cancer cell lines such as MCF-7 (breast cancer) and HCT116 (colon cancer) revealed that this compound exhibits cytotoxic effects with IC50 values indicating significant cell death at certain concentrations .
Data Tables
Biological Activity | Assay Method | IC50 Value |
---|---|---|
Antioxidant Activity | DPPH | 4.36 ± 0.91 mg/mL |
Antioxidant Activity | ABTS | 2.12 ± 0.05 mg/mL |
Cytotoxicity | MTT Assay | Varies by cell line |
Case Study 1: Antioxidant Evaluation
A detailed study analyzed the antioxidant capacity of various aldehydes including this compound. The results indicated that while it showed moderate activity, it was less effective than standard antioxidants like Trolox and BHT .
Case Study 2: Antimicrobial Efficacy
In a comparative study on the antimicrobial properties of several organic compounds, this compound was tested against common foodborne pathogens such as E. coli and Salmonella spp. The findings confirmed its effectiveness in inhibiting bacterial growth at specific concentrations .
Q & A
Basic Research Questions
Q. What experimental strategies are recommended for synthesizing 3,3-Dimethylhexanal with high purity and yield?
- Methodological Answer : Optimize reaction conditions using catalytic hydrogenation or oxidation of 3,3-dimethylhexanol. Monitor reaction progress via gas chromatography (GC) to track intermediates and final product purity . Thermochemical data (e.g., enthalpy of vaporization) from NIST can guide solvent selection and distillation parameters . Validate purity using spectroscopic techniques (e.g., NMR, IR) and compare retention indices with reference databases .
Q. How can researchers characterize the structural and physicochemical properties of this compound?
- Methodological Answer : Use computational tools (e.g., ACD/Labs Percepta Platform) to predict properties like logP, pKa, and solubility . Experimentally validate via differential scanning calorimetry (DSC) for melting points and dynamic vapor sorption (DVS) for hygroscopicity. Cross-reference with PubChem or CAS Common Chemistry for structural verification .
Q. What safety protocols should be prioritized when handling this compound in laboratory settings?
- Methodological Answer : Follow OSHA and ECHA guidelines for aldehydes: use fume hoods, wear nitrile gloves, and avoid ignition sources. Conduct a hazard assessment using Safety Data Sheets (SDS) for structurally similar compounds (e.g., 3,3'-Diaminobenzidine) to infer risks like flammability or toxicity .
Advanced Research Questions
Q. How can contradictory data on this compound’s reactivity be resolved in cross-disciplinary studies?
- Methodological Answer : Perform systematic kinetic studies under controlled conditions (temperature, solvent polarity) to isolate variables. Use density functional theory (DFT) calculations to model reaction pathways and compare with experimental outcomes . Cross-validate results using databases like NIST Chemistry WebBook for thermochemical consistency .
Q. What advanced techniques are suitable for probing this compound’s role in catalytic cycles or reaction mechanisms?
- Methodological Answer : Employ isotopically labeled analogs (e.g., deuterated derivatives) in kinetic isotope effect (KIE) studies. Use in situ FTIR or Raman spectroscopy to monitor intermediate formation. Reference synthetic methodologies from diacetylenic diamine studies to design analogous reaction templates .
Q. How can researchers design in vitro assays to evaluate this compound’s biological activity without reliable prior data?
- Methodological Answer : Adopt structure-activity relationship (SAR) models based on analogs like 3,3-Dimethylcyclohexanamine hydrochloride. Test for anti-inflammatory activity via COX-2/iNOS inhibition assays in macrophage cell lines, ensuring proper controls (e.g., solvent-only blanks) . Validate cytotoxicity using MTT assays and compare with PubChem bioactivity data .
Q. What computational approaches are effective in predicting this compound’s environmental fate or toxicological profile?
- Methodological Answer : Use EPI Suite or ChemAxon tools to estimate biodegradation half-lives and bioaccumulation potential. Validate with Daphnia magna or algal toxicity assays, referencing ECHA ecological hazard classifications for structurally related aldehydes .
Q. Methodological Notes
- Data Validation : Cross-reference experimental results with authoritative databases (e.g., PubChem, NIST) to resolve discrepancies .
- Synthetic Optimization : Prioritize green chemistry principles (e.g., solvent-free reactions) to align with EPA and REACH sustainability criteria .
- Risk Mitigation : Conduct small-scale pilot studies before scaling reactions, adhering to Seveso III Directive thresholds for hazardous substances .
Properties
IUPAC Name |
3,3-dimethylhexanal | |
---|---|---|
Details | Computed by Lexichem TK 2.7.0 (PubChem release 2021.10.14) | |
Source | PubChem | |
URL | https://pubchem.ncbi.nlm.nih.gov | |
Description | Data deposited in or computed by PubChem | |
InChI |
InChI=1S/C8H16O/c1-4-5-8(2,3)6-7-9/h7H,4-6H2,1-3H3 | |
Details | Computed by InChI 1.0.6 (PubChem release 2021.10.14) | |
Source | PubChem | |
URL | https://pubchem.ncbi.nlm.nih.gov | |
Description | Data deposited in or computed by PubChem | |
InChI Key |
IHEORNQYISDNQZ-UHFFFAOYSA-N | |
Details | Computed by InChI 1.0.6 (PubChem release 2021.10.14) | |
Source | PubChem | |
URL | https://pubchem.ncbi.nlm.nih.gov | |
Description | Data deposited in or computed by PubChem | |
Canonical SMILES |
CCCC(C)(C)CC=O | |
Details | Computed by OEChem 2.3.0 (PubChem release 2021.10.14) | |
Source | PubChem | |
URL | https://pubchem.ncbi.nlm.nih.gov | |
Description | Data deposited in or computed by PubChem | |
Molecular Formula |
C8H16O | |
Details | Computed by PubChem 2.2 (PubChem release 2021.10.14) | |
Source | PubChem | |
URL | https://pubchem.ncbi.nlm.nih.gov | |
Description | Data deposited in or computed by PubChem | |
DSSTOX Substance ID |
DTXSID70339758 | |
Record name | 3,3-Dimethylhexanal | |
Source | EPA DSSTox | |
URL | https://comptox.epa.gov/dashboard/DTXSID70339758 | |
Description | DSSTox provides a high quality public chemistry resource for supporting improved predictive toxicology. | |
Molecular Weight |
128.21 g/mol | |
Details | Computed by PubChem 2.2 (PubChem release 2021.10.14) | |
Source | PubChem | |
URL | https://pubchem.ncbi.nlm.nih.gov | |
Description | Data deposited in or computed by PubChem | |
CAS No. |
55320-57-5 | |
Record name | 3,3-Dimethylhexanal | |
Source | EPA DSSTox | |
URL | https://comptox.epa.gov/dashboard/DTXSID70339758 | |
Description | DSSTox provides a high quality public chemistry resource for supporting improved predictive toxicology. | |
Disclaimer and Information on In-Vitro Research Products
Please be aware that all articles and product information presented on BenchChem are intended solely for informational purposes. The products available for purchase on BenchChem are specifically designed for in-vitro studies, which are conducted outside of living organisms. In-vitro studies, derived from the Latin term "in glass," involve experiments performed in controlled laboratory settings using cells or tissues. It is important to note that these products are not categorized as medicines or drugs, and they have not received approval from the FDA for the prevention, treatment, or cure of any medical condition, ailment, or disease. We must emphasize that any form of bodily introduction of these products into humans or animals is strictly prohibited by law. It is essential to adhere to these guidelines to ensure compliance with legal and ethical standards in research and experimentation.