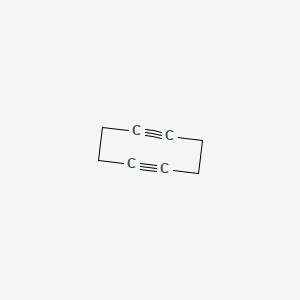
1,5-Cyclooctadiyne
- Click on QUICK INQUIRY to receive a quote from our team of experts.
- With the quality product at a COMPETITIVE price, you can focus more on your research.
Overview
Description
1,5-Cyclooctadiyne is a cyclic hydrocarbon with the chemical formula C8H8. It is characterized by the presence of two triple bonds within an eight-membered ring structure. This compound is known for its high strain energy due to the presence of these triple bonds, making it a highly reactive molecule.
Preparation Methods
1,5-Cyclooctadiyne can be synthesized through various methods. One common synthetic route involves the dimerization of butadiene in the presence of a nickel catalyst. This reaction also produces vinylcyclohexene as a byproduct . Another method involves the reaction of potassium tetrachloroplatinate with 1,5-cyclooctadiene in the presence of a phase transfer catalyst such as PEG-400. This reaction is carried out in a mixed solution of n-propyl alcohol and water at 50°C for 4 hours, yielding dichloro (1,5-cyclooctadiene) platinum (II) with a high yield .
Chemical Reactions Analysis
1,5-Cyclooctadiyne undergoes a variety of chemical reactions due to its highly strained structure. Some of the common reactions include:
Oxidation: The compound can be oxidized to form various oxygenated derivatives.
Reduction: Reduction reactions can convert the triple bonds into double or single bonds, leading to the formation of cyclooctadiene or cyclooctane.
Substitution: Nucleophilic substitution reactions can introduce various functional groups into the molecule.
Cycloaddition: The compound can undergo [3+2] cycloadditions with 1,3-dipoles, forming cycloadducts at a rate comparable to strained cyclooctynes.
Common reagents used in these reactions include borane, sulfur dichloride, and various nucleophiles. The major products formed from these reactions depend on the specific conditions and reagents used.
Scientific Research Applications
1,5-Cyclooctadiyne has numerous applications in scientific research, particularly in the fields of chemistry and materials science. Some of its notable applications include:
Click Chemistry: The compound is used in click chemistry reactions due to its ability to undergo rapid and efficient cycloadditions.
Ligand in Organometallic Chemistry: It serves as a ligand in the formation of various organometallic complexes, which are used as catalysts in organic synthesis.
Polymer Chemistry: The compound is used in the synthesis of novel polymers with unique properties.
Bioconjugation: Its reactivity makes it useful in bioconjugation reactions, where it is used to link biomolecules for various applications in biology and medicine.
Mechanism of Action
The mechanism of action of 1,5-cyclooctadiyne involves its high strain energy, which makes it highly reactive. The compound can undergo various cycloaddition reactions, forming stable cycloadducts. These reactions often involve the formation of intermediate species, such as biradicals or carbocations, which then undergo further transformations to yield the final products .
Comparison with Similar Compounds
1,5-Cyclooctadiyne can be compared to other cyclic hydrocarbons with multiple bonds, such as 1,5-cyclooctadiene and 1,3-cyclooctadiene. While 1,5-cyclooctadiene contains two double bonds and is less strained, this compound contains two triple bonds, making it more reactive and suitable for different types of chemical reactions . Other similar compounds include cyclooctyne and cyclooctatetraene, which also exhibit unique reactivity due to their strained ring structures.
Properties
IUPAC Name |
cycloocta-1,5-diyne |
Source
|
---|---|---|
Details | Computed by Lexichem TK 2.7.0 (PubChem release 2021.10.14) | |
Source | PubChem | |
URL | https://pubchem.ncbi.nlm.nih.gov | |
Description | Data deposited in or computed by PubChem | |
InChI |
InChI=1S/C8H8/c1-2-4-6-8-7-5-3-1/h1-2,7-8H2 |
Source
|
Details | Computed by InChI 1.0.6 (PubChem release 2021.10.14) | |
Source | PubChem | |
URL | https://pubchem.ncbi.nlm.nih.gov | |
Description | Data deposited in or computed by PubChem | |
InChI Key |
URBAEOVIKFSRNB-UHFFFAOYSA-N |
Source
|
Details | Computed by InChI 1.0.6 (PubChem release 2021.10.14) | |
Source | PubChem | |
URL | https://pubchem.ncbi.nlm.nih.gov | |
Description | Data deposited in or computed by PubChem | |
Canonical SMILES |
C1CC#CCCC#C1 |
Source
|
Details | Computed by OEChem 2.3.0 (PubChem release 2021.10.14) | |
Source | PubChem | |
URL | https://pubchem.ncbi.nlm.nih.gov | |
Description | Data deposited in or computed by PubChem | |
Molecular Formula |
C8H8 |
Source
|
Details | Computed by PubChem 2.2 (PubChem release 2021.10.14) | |
Source | PubChem | |
URL | https://pubchem.ncbi.nlm.nih.gov | |
Description | Data deposited in or computed by PubChem | |
DSSTOX Substance ID |
DTXSID70198125 |
Source
|
Record name | 1,5-Cyclooctadiyne | |
Source | EPA DSSTox | |
URL | https://comptox.epa.gov/dashboard/DTXSID70198125 | |
Description | DSSTox provides a high quality public chemistry resource for supporting improved predictive toxicology. | |
Molecular Weight |
104.15 g/mol |
Source
|
Details | Computed by PubChem 2.2 (PubChem release 2021.10.14) | |
Source | PubChem | |
URL | https://pubchem.ncbi.nlm.nih.gov | |
Description | Data deposited in or computed by PubChem | |
CAS No. |
49852-40-6 |
Source
|
Record name | 1,5-Cyclooctadiyne | |
Source | ChemIDplus | |
URL | https://pubchem.ncbi.nlm.nih.gov/substance/?source=chemidplus&sourceid=0049852406 | |
Description | ChemIDplus is a free, web search system that provides access to the structure and nomenclature authority files used for the identification of chemical substances cited in National Library of Medicine (NLM) databases, including the TOXNET system. | |
Record name | 1,5-Cyclooctadiyne | |
Source | EPA DSSTox | |
URL | https://comptox.epa.gov/dashboard/DTXSID70198125 | |
Description | DSSTox provides a high quality public chemistry resource for supporting improved predictive toxicology. | |
Disclaimer and Information on In-Vitro Research Products
Please be aware that all articles and product information presented on BenchChem are intended solely for informational purposes. The products available for purchase on BenchChem are specifically designed for in-vitro studies, which are conducted outside of living organisms. In-vitro studies, derived from the Latin term "in glass," involve experiments performed in controlled laboratory settings using cells or tissues. It is important to note that these products are not categorized as medicines or drugs, and they have not received approval from the FDA for the prevention, treatment, or cure of any medical condition, ailment, or disease. We must emphasize that any form of bodily introduction of these products into humans or animals is strictly prohibited by law. It is essential to adhere to these guidelines to ensure compliance with legal and ethical standards in research and experimentation.