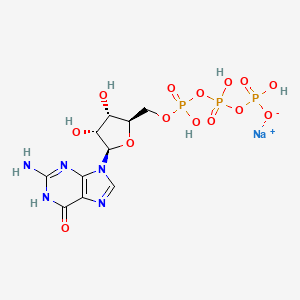
5'-GTP Sodium Salt; Sodium GTP
- Click on QUICK INQUIRY to receive a quote from our team of experts.
- With the quality product at a COMPETITIVE price, you can focus more on your research.
Overview
Description
5’-Guanosine Triphosphate Sodium Salt, commonly referred to as Sodium GTP, is a purine trinucleotide. It plays a crucial role in various biochemical processes, including signal transduction, protein synthesis, and as a substrate for RNA synthesis during transcription . Sodium GTP is a key molecule in cellular energy transfer and is involved in numerous cellular functions.
Preparation Methods
Synthetic Routes and Reaction Conditions: Sodium GTP is typically prepared by the enzymatic phosphorylation of guanosine 5’-monophosphate (GMP). This process involves the addition of phosphate groups to GMP, facilitated by specific enzymes . The compound is then purified using ion exchange chromatography to ensure high purity and quality .
Industrial Production Methods: In industrial settings, the production of Sodium GTP follows similar enzymatic processes but on a larger scale. The enzymatic phosphorylation is optimized for higher yields, and advanced purification techniques are employed to meet industrial standards .
Chemical Reactions Analysis
Types of Reactions: Sodium GTP undergoes various chemical reactions, including hydrolysis, phosphorylation, and interactions with proteins. It is involved in the hydrolysis reaction where it is converted to guanosine diphosphate (GDP) and inorganic phosphate .
Common Reagents and Conditions:
Hydrolysis: Catalyzed by GTPases, resulting in the formation of GDP and inorganic phosphate.
Phosphorylation: Involves kinases that add phosphate groups to the molecule.
Protein Interactions: Sodium GTP binds to G-proteins, influencing their activity and function.
Major Products Formed:
GDP (Guanosine Diphosphate): Formed during hydrolysis.
GTP-bound Proteins: Formed during interactions with G-proteins.
Scientific Research Applications
Sodium GTP has a wide range of applications in scientific research:
Mechanism of Action
Sodium GTP functions as a carrier of phosphates and pyrophosphates, channeling chemical energy into specific biosynthetic pathways . It activates signal transducing G-proteins, which are involved in various cellular processes, including proliferation, differentiation, and activation of intracellular kinase cascades . The hydrolysis of Sodium GTP by small GTPases such as Ras and Rho regulates proliferation and apoptosis .
Comparison with Similar Compounds
Adenosine Triphosphate (ATP): Another nucleotide involved in energy transfer.
Cytidine Triphosphate (CTP): Used in the synthesis of RNA.
Uridine Triphosphate (UTP): Involved in carbohydrate metabolism.
Uniqueness of Sodium GTP: Sodium GTP is unique due to its specific role in G-protein signaling and its involvement in the regulation of various cellular processes. Unlike ATP, which is primarily an energy currency, Sodium GTP has specialized functions in signal transduction and protein synthesis .
Properties
Molecular Formula |
C10H15N5NaO14P3 |
---|---|
Molecular Weight |
545.16 g/mol |
IUPAC Name |
sodium;[[[(2R,3S,4R,5R)-5-(2-amino-6-oxo-1H-purin-9-yl)-3,4-dihydroxyoxolan-2-yl]methoxy-hydroxyphosphoryl]oxy-hydroxyphosphoryl] hydrogen phosphate |
InChI |
InChI=1S/C10H16N5O14P3.Na/c11-10-13-7-4(8(18)14-10)12-2-15(7)9-6(17)5(16)3(27-9)1-26-31(22,23)29-32(24,25)28-30(19,20)21;/h2-3,5-6,9,16-17H,1H2,(H,22,23)(H,24,25)(H2,19,20,21)(H3,11,13,14,18);/q;+1/p-1/t3-,5-,6-,9-;/m1./s1 |
InChI Key |
XZLBHDBHXGWQOB-GWTDSMLYSA-M |
Isomeric SMILES |
C1=NC2=C(N1[C@H]3[C@@H]([C@@H]([C@H](O3)COP(=O)(O)OP(=O)(O)OP(=O)(O)[O-])O)O)N=C(NC2=O)N.[Na+] |
Canonical SMILES |
C1=NC2=C(N1C3C(C(C(O3)COP(=O)(O)OP(=O)(O)OP(=O)(O)[O-])O)O)N=C(NC2=O)N.[Na+] |
Origin of Product |
United States |
Disclaimer and Information on In-Vitro Research Products
Please be aware that all articles and product information presented on BenchChem are intended solely for informational purposes. The products available for purchase on BenchChem are specifically designed for in-vitro studies, which are conducted outside of living organisms. In-vitro studies, derived from the Latin term "in glass," involve experiments performed in controlled laboratory settings using cells or tissues. It is important to note that these products are not categorized as medicines or drugs, and they have not received approval from the FDA for the prevention, treatment, or cure of any medical condition, ailment, or disease. We must emphasize that any form of bodily introduction of these products into humans or animals is strictly prohibited by law. It is essential to adhere to these guidelines to ensure compliance with legal and ethical standards in research and experimentation.