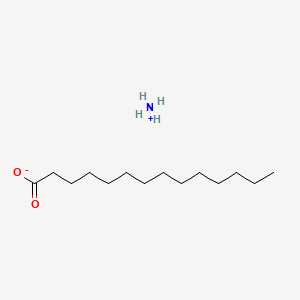
Ammonium myristate
- Click on QUICK INQUIRY to receive a quote from our team of experts.
- With the quality product at a COMPETITIVE price, you can focus more on your research.
Overview
Description
Ammonium myristate is a chemical compound with the molecular formula C14H31NO2. It is the ammonium salt of myristic acid, a common saturated fatty acid. This compound is often used in various industrial and scientific applications due to its unique properties and behavior.
Preparation Methods
Synthetic Routes and Reaction Conditions: Ammonium myristate can be synthesized by neutralizing myristic acid with ammonium hydroxide. The reaction typically involves dissolving myristic acid in an alcoholic solution and then adding concentrated ammonium hydroxide. The mixture is then allowed to crystallize under controlled conditions .
Industrial Production Methods: In industrial settings, the preparation of this compound often involves large-scale neutralization processes. The myristic acid is dissolved in a suitable solvent, and ammonium hydroxide is added gradually. The resulting solution is then subjected to crystallization and purification processes to obtain high-purity this compound .
Chemical Reactions Analysis
Types of Reactions: Ammonium myristate can undergo various chemical reactions, including:
Oxidation: It can be oxidized to form myristic acid and other oxidation products.
Reduction: Reduction reactions can convert it back to myristic acid.
Substitution: It can participate in substitution reactions where the ammonium ion is replaced by other cations.
Common Reagents and Conditions:
Oxidation: Common oxidizing agents include potassium permanganate and hydrogen peroxide.
Reduction: Reducing agents such as lithium aluminum hydride can be used.
Substitution: Reagents like sodium hydroxide can facilitate substitution reactions.
Major Products: The major products formed from these reactions include myristic acid, various myristate salts, and other derivatives depending on the specific reaction conditions .
Scientific Research Applications
Ammonium myristate has a wide range of applications in scientific research:
Chemistry: It is used as a surfactant and emulsifying agent in various chemical formulations.
Biology: It plays a role in studies involving lipid metabolism and membrane biology.
Medicine: It is used in the formulation of certain pharmaceuticals and cosmetic products.
Industry: It is utilized in the production of soaps, detergents, and other personal care products.
Mechanism of Action
The mechanism of action of ammonium myristate involves its interaction with lipid membranes and proteins. It can integrate into lipid bilayers, affecting membrane fluidity and permeability. Additionally, it can interact with specific proteins, influencing their function and activity. These interactions are crucial in its applications as a surfactant and emulsifying agent .
Comparison with Similar Compounds
Ammonium Palmitate: Similar in structure but with a longer carbon chain.
Ammonium Stearate: Another similar compound with an even longer carbon chain.
Ammonium Laurate: Has a shorter carbon chain compared to ammonium myristate.
Uniqueness: this compound is unique due to its specific chain length, which provides a balance between hydrophobic and hydrophilic properties. This balance makes it particularly effective as a surfactant and emulsifying agent in various applications .
Properties
CAS No. |
16530-71-5 |
---|---|
Molecular Formula |
C14H28O2.H3N C14H31NO2 |
Molecular Weight |
245.40 g/mol |
IUPAC Name |
azanium;tetradecanoate |
InChI |
InChI=1S/C14H28O2.H3N/c1-2-3-4-5-6-7-8-9-10-11-12-13-14(15)16;/h2-13H2,1H3,(H,15,16);1H3 |
InChI Key |
BJOYVZZDDFVLPI-UHFFFAOYSA-N |
Canonical SMILES |
CCCCCCCCCCCCCC(=O)[O-].[NH4+] |
Origin of Product |
United States |
Disclaimer and Information on In-Vitro Research Products
Please be aware that all articles and product information presented on BenchChem are intended solely for informational purposes. The products available for purchase on BenchChem are specifically designed for in-vitro studies, which are conducted outside of living organisms. In-vitro studies, derived from the Latin term "in glass," involve experiments performed in controlled laboratory settings using cells or tissues. It is important to note that these products are not categorized as medicines or drugs, and they have not received approval from the FDA for the prevention, treatment, or cure of any medical condition, ailment, or disease. We must emphasize that any form of bodily introduction of these products into humans or animals is strictly prohibited by law. It is essential to adhere to these guidelines to ensure compliance with legal and ethical standards in research and experimentation.