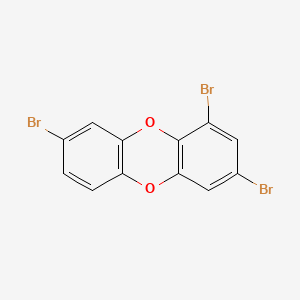
1,3,8-Tribromodibenzo-P-dioxin
- Click on QUICK INQUIRY to receive a quote from our team of experts.
- With the quality product at a COMPETITIVE price, you can focus more on your research.
Overview
Description
1,3,8-Tribromodibenzo-P-dioxin is a polybrominated dibenzo-p-dioxin (PBDD) compound. It is a derivative of dibenzo-p-dioxin, where three hydrogen atoms are replaced by bromine atoms at positions 1, 3, and 8. This compound is of significant interest due to its potential environmental and health impacts, as well as its role in various chemical processes.
Preparation Methods
1,3,8-Tribromodibenzo-P-dioxin can be synthesized through the oxidation of hydroxylated polybrominated diphenyl ethers (OH-PBDEs) using iron and manganese oxides under dry conditions . The transformation involves the use of goethite and manganese oxides, which facilitate the conversion of OH-PBDEs to this compound and other related compounds . The reaction conditions typically include a controlled environment to prevent the presence of water, as water inhibits the formation of PBDDs .
Chemical Reactions Analysis
1,3,8-Tribromodibenzo-P-dioxin undergoes various chemical reactions, including:
Oxidation: The compound can be formed through the oxidation of OH-PBDEs using iron and manganese oxides.
Photolysis: Under UV irradiation, hydroxylated polybromodiphenyl ethers can degrade to form this compound.
Common reagents used in these reactions include iron and manganese oxides, UV light, and hydrogen peroxide. The major products formed from these reactions are other polybrominated dibenzo-p-dioxins and dibenzofurans .
Scientific Research Applications
1,3,8-Tribromodibenzo-P-dioxin is studied extensively in environmental science and toxicology due to its potential as an endocrine disruptor and its persistence in the environment . It is also used as a model compound to understand the formation and behavior of polybrominated dibenzo-p-dioxins and dibenzofurans in various environmental processes . Additionally, its formation and degradation pathways are investigated to develop methods for mitigating its presence in the environment .
Mechanism of Action
The mechanism by which 1,3,8-Tribromodibenzo-P-dioxin exerts its effects involves its interaction with various molecular targets. It can bind to human transthyretin (TTR) due to its structural similarity to thyroid hormones . This binding can disrupt normal hormonal functions, leading to endocrine disruption. The compound’s formation involves Smiles rearrangements and bromine elimination processes .
Comparison with Similar Compounds
1,3,8-Tribromodibenzo-P-dioxin is similar to other polybrominated dibenzo-p-dioxins and dibenzofurans, such as 2,4,6,8-tetrabromodibenzofuran . These compounds share similar structures and formation pathways but differ in the number and positions of bromine atoms. The unique aspect of this compound is its specific bromination pattern, which influences its chemical behavior and toxicity.
Similar compounds include:
- 2,4,6,8-Tetrabromodibenzofuran
- 2,7-Dibromodibenzo-p-dioxin
- 2,4,6-Tribromophenol
These compounds are studied to understand the broader category of polybrominated dibenzo-p-dioxins and their environmental and health impacts.
Biological Activity
1,3,8-Tribromodibenzo-P-dioxin (1,3,8-TrBDD) is a halogenated aromatic compound belonging to the family of dibenzo-p-dioxins. It has garnered attention due to its potential environmental persistence and biological activity. This article aims to explore the biological activity of 1,3,8-TrBDD, including its synthesis, metabolic pathways, effects on living organisms, and ecological implications.
Synthesis and Formation
1,3,8-TrBDD can be formed through various chemical reactions, particularly photolysis of polybrominated diphenyl ethers (PBDEs). Research indicates that it is produced exclusively via direct photolysis under specific conditions. The formation pathways include the cleavage of ether bonds in PBDEs, leading to the generation of dioxin compounds .
Toxicological Profile
The toxicological effects of 1,3,8-TrBDD have not been as extensively studied as those of other dioxins like TCDD (2,3,7,8-tetrachlorodibenzo-p-dioxin). However, preliminary studies suggest that it may exhibit similar biological activities:
- Enzyme Induction : Like TCDD, 1,3,8-TrBDD may induce cytochrome P450 enzymes. These enzymes are crucial for the metabolism of xenobiotics and can lead to the production of reactive metabolites that contribute to toxicity .
- Cellular Effects : Studies on related compounds indicate that exposure can lead to alterations in cellular processes such as apoptosis and cellular proliferation. For instance, TCDD has been shown to affect gene expression through the aryl hydrocarbon receptor (AhR) pathway .
Case Studies
- Aquatic Toxicity : A study demonstrated that 1,3,8-TrBDD could impact aquatic organisms by inducing oxidative stress and altering reproductive functions. The research highlighted its potential role as an endocrine disruptor in fish species .
- Mammalian Models : In rodent models exposed to various dioxins including TrBDD analogs, significant changes in liver enzyme activities were observed. These changes are indicative of hepatic stress and potential carcinogenic effects .
Comparative Biological Activity
To better understand the biological activity of 1,3,8-TrBDD relative to other dioxins, a comparison table is provided below:
Environmental Implications
The persistence of 1,3,8-TrBDD in the environment raises concerns regarding its ecological impact. As a product of PBDE degradation and photolytic processes in aquatic systems, it poses risks not only to wildlife but also potentially to human health through bioaccumulation in food chains .
Properties
CAS No. |
80246-33-9 |
---|---|
Molecular Formula |
C12H5Br3O2 |
Molecular Weight |
420.88 g/mol |
IUPAC Name |
1,3,8-tribromodibenzo-p-dioxin |
InChI |
InChI=1S/C12H5Br3O2/c13-6-1-2-9-10(4-6)17-12-8(15)3-7(14)5-11(12)16-9/h1-5H |
InChI Key |
NLKFBKPFQWQPPL-UHFFFAOYSA-N |
Canonical SMILES |
C1=CC2=C(C=C1Br)OC3=C(O2)C=C(C=C3Br)Br |
Origin of Product |
United States |
Disclaimer and Information on In-Vitro Research Products
Please be aware that all articles and product information presented on BenchChem are intended solely for informational purposes. The products available for purchase on BenchChem are specifically designed for in-vitro studies, which are conducted outside of living organisms. In-vitro studies, derived from the Latin term "in glass," involve experiments performed in controlled laboratory settings using cells or tissues. It is important to note that these products are not categorized as medicines or drugs, and they have not received approval from the FDA for the prevention, treatment, or cure of any medical condition, ailment, or disease. We must emphasize that any form of bodily introduction of these products into humans or animals is strictly prohibited by law. It is essential to adhere to these guidelines to ensure compliance with legal and ethical standards in research and experimentation.