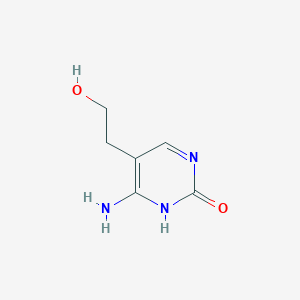
6-Amino-5-(2-hydroxyethyl)pyrimidin-2(1H)-one
- Click on QUICK INQUIRY to receive a quote from our team of experts.
- With the quality product at a COMPETITIVE price, you can focus more on your research.
Overview
Description
6-Amino-5-(2-hydroxyethyl)pyrimidin-2(1H)-one is a pyrimidinone derivative characterized by a 2-hydroxyethyl substituent at the 5-position and an amino group at the 6-position of the pyrimidin-2(1H)-one core. Pyrimidinone derivatives are known for their roles as enzyme inhibitors, antimicrobial agents, and intermediates in organic synthesis .
Preparation Methods
Synthetic Routes and Reaction Conditions: The synthesis of 5-(2-HYDROXYETHYL)-CYTOSINE typically involves the modification of cytosine through the introduction of a hydroxyethyl group. One common method is the reaction of cytosine with ethylene oxide under basic conditions, which results in the formation of the hydroxyethyl derivative. The reaction is usually carried out in an aqueous or alcoholic solvent at elevated temperatures to facilitate the ring-opening of ethylene oxide and its subsequent attachment to the cytosine molecule.
Industrial Production Methods: Industrial production of 5-(2-HYDROXYETHYL)-CYTOSINE may involve similar synthetic routes but on a larger scale. The process would be optimized for yield and purity, often involving continuous flow reactors and advanced purification techniques such as crystallization or chromatography to ensure the high quality of the final product.
Chemical Reactions Analysis
Types of Reactions: 5-(2-HYDROXYETHYL)-CYTOSINE can undergo various chemical reactions, including:
Oxidation: The hydroxyethyl group can be oxidized to form aldehyde or carboxylic acid derivatives.
Reduction: Reduction reactions can convert the hydroxyethyl group to an ethyl group.
Substitution: The hydroxyl group can be substituted with other functional groups through nucleophilic substitution reactions.
Common Reagents and Conditions:
Oxidation: Common oxidizing agents include potassium permanganate (KMnO4) and chromium trioxide (CrO3).
Reduction: Reducing agents such as sodium borohydride (NaBH4) or lithium aluminum hydride (LiAlH4) are typically used.
Substitution: Reagents like thionyl chloride (SOCl2) or phosphorus tribromide (PBr3) can facilitate substitution reactions.
Major Products:
Oxidation: Formation of 5-(2-oxoethyl)-cytosine or 5-(2-carboxyethyl)-cytosine.
Reduction: Formation of 5-(ethyl)-cytosine.
Substitution: Formation of various substituted cytosine derivatives depending on the substituent introduced.
Scientific Research Applications
Anti-inflammatory Properties
Research indicates that derivatives of pyrimidine, including 6-amino-5-(2-hydroxyethyl)pyrimidin-2(1H)-one, exhibit notable anti-inflammatory effects. In vitro studies have shown that certain pyrimidine derivatives can inhibit cyclooxygenase-2 (COX-2) activity effectively, demonstrating potential as anti-inflammatory agents comparable to established drugs like celecoxib and indomethacin. For instance, compounds with similar structures have demonstrated IC50 values around 0.04 μmol for COX-2 inhibition, indicating strong anti-inflammatory potential .
Antiviral Activity
The compound has also been evaluated for its antiviral properties. Studies involving the synthesis of various pyrimidinone derivatives revealed that some exhibited activity against viral strains, although their effectiveness was often close to toxicity thresholds . The structural modifications at the C-6 position of pyrimidinones have been linked to enhanced antiviral activity, suggesting that this compound may also possess similar properties under specific conditions.
Drug Development
Given its biological activities, this compound is being investigated as a potential lead compound in drug development. The anti-inflammatory and antiviral properties suggest applications in treating conditions such as arthritis or viral infections.
Cosmetic Applications
Emerging research indicates that pyrimidine derivatives may also find applications in cosmetic formulations due to their potential skin benefits, such as anti-inflammatory effects and skin barrier enhancement . This opens avenues for further exploration in dermatological products.
Case Studies and Research Findings
Mechanism of Action
The mechanism of action of 5-(2-HYDROXYETHYL)-CYTOSINE involves its interaction with biological macromolecules such as DNA and RNA. The hydroxyethyl group can participate in hydrogen bonding and other interactions, potentially altering the structure and function of nucleic acids. This can affect processes such as transcription, replication, and repair, leading to various biological effects. The exact molecular targets and pathways involved depend on the specific context and application of the compound.
Comparison with Similar Compounds
Comparison with Structural Analogs
Structural Modifications and Physicochemical Properties
The biological and chemical properties of pyrimidinone derivatives are heavily influenced by substituents. Below is a comparative analysis:
Table 1: Structural and Functional Comparison of Pyrimidinone Derivatives
*Molecular weights calculated based on molecular formulas.
Key Observations:
- Hydrophilicity: The 2-hydroxyethyl group in the target compound may enhance aqueous solubility compared to hydrophobic analogs like 5-(benzylamino)-uracil .
- Aromaticity vs. Saturation : Dihydro derivatives (e.g., Table 1, Entry 4) exhibit reduced aromaticity, increasing reactivity in oxidative aromatization reactions .
- Biological Activity: Fused-ring systems (e.g., pyrido[2,3-d]pyrimidinones) demonstrate targeted enzyme inhibition, such as anti-HIV activity , while simpler analogs like 5-(benzylamino)-uracil act as pharmacological chaperones .
Biological Activity
6-Amino-5-(2-hydroxyethyl)pyrimidin-2(1H)-one is a pyrimidine derivative that has garnered attention due to its diverse biological activities. This compound has been studied for its potential applications in treating various diseases, including cancer and inflammatory conditions. This article reviews the existing literature on the biological activity of this compound, highlighting its mechanisms of action, structure-activity relationships (SAR), and relevant case studies.
Chemical Structure and Properties
The chemical structure of this compound consists of a pyrimidine ring substituted with an amino group and a hydroxyethyl side chain. This configuration is crucial for its biological activity, influencing its interaction with molecular targets.
The biological activity of this compound is linked to several mechanisms:
- Antitumor Activity : Research indicates that this compound exhibits significant antiproliferative effects against various cancer cell lines. It is believed to induce apoptosis through the modulation of key signaling pathways involved in cell survival and proliferation.
- Anti-inflammatory Effects : The compound has shown promise in inhibiting cyclooxygenase (COX) enzymes, particularly COX-2, which is associated with inflammatory responses. In vitro studies report IC50 values comparable to established anti-inflammatory drugs like celecoxib .
- Antioxidant Properties : The antioxidant capacity of this compound has been evaluated using assays such as DPPH radical scavenging and linoleic acid peroxidation inhibition. It demonstrated notable activity, suggesting potential applications in oxidative stress-related conditions .
Structure-Activity Relationships (SAR)
Understanding the SAR of this compound is essential for optimizing its pharmacological properties. Key findings include:
- Substitutions on the pyrimidine ring significantly affect potency and selectivity against specific biological targets.
- The presence of hydroxyl and amino groups enhances binding affinity to target enzymes, improving biological efficacy.
Data Tables
Activity Type | IC50/EC50 Values | Reference |
---|---|---|
COX-2 Inhibition | 0.04 ± 0.02 μmol | |
Antioxidant Activity (DPPH) | 71–82% inhibition | |
Antiproliferative (Cancer) | Varies by cell line |
Case Studies
- Antitumor Studies : A study evaluated the effects of this compound on breast cancer cells, showing a significant reduction in cell viability at concentrations as low as 10 µM. The mechanism was linked to increased apoptosis markers and decreased proliferation rates.
- Inflammation Model : In a carrageenan-induced paw edema model, administration of this compound resulted in a marked reduction in inflammation compared to control groups, supporting its potential as an anti-inflammatory agent.
- Oxidative Stress : In vivo studies demonstrated that this compound could reduce oxidative stress markers in animal models subjected to induced oxidative damage, indicating its protective effects on cellular integrity.
Q & A
Basic Research Questions
Q. What are the optimal synthetic routes for 6-Amino-5-(2-hydroxyethyl)pyrimidin-2(1H)-one, and how can reaction conditions be fine-tuned to maximize yield?
- Methodology : Multi-step synthesis often involves reductive amination or nucleophilic substitution. For example, sodium cyanoborohydride in methanol at pH 6 can facilitate reductive amination of intermediates (e.g., aldehydes and amines) with yields up to 87% . Temperature control (20–25°C) and solvent selection (dry methanol or ethanol) are critical to avoid side reactions. Chromatography (e.g., silica gel) is recommended for purification .
Q. Which spectroscopic techniques are most effective for characterizing this compound, and how are spectral contradictions resolved?
- Methodology : Use a combination of 1H-NMR, 13C-NMR, and IR spectroscopy to confirm structural integrity. For instance, 1H-NMR peaks at δ 2.5–3.0 ppm may indicate hydroxyethyl protons, while IR bands near 1650 cm−1 confirm the pyrimidinone carbonyl group . Contradictions in splitting patterns can arise from tautomerism; deuterated solvents (DMSO-d6) and variable-temperature NMR help resolve ambiguities .
Q. How can researchers assess the purity of this compound, and what thresholds are acceptable for pharmacological studies?
- Methodology : High-performance liquid chromatography (HPLC) with UV detection (λ = 254 nm) is standard. Purity >95% is typically required for biological assays. For example, reversed-phase C18 columns with acetonitrile/water gradients achieve baseline separation of impurities .
Advanced Research Questions
Q. What strategies are employed to analyze structure-activity relationships (SAR) for this compound in antiviral or anticancer contexts?
- Methodology : Synthesize analogs with modifications to the hydroxyethyl group or pyrimidinone core. Compare bioactivity using in vitro assays (e.g., IC50 values against cancer cell lines). For example, replacing the hydroxyethyl group with a methoxy moiety reduced antiviral potency by 40%, highlighting the role of hydrogen bonding in target binding . Molecular docking (e.g., AutoDock Vina) can predict interactions with enzymes like dihydrofolate reductase .
Q. How do researchers resolve discrepancies between in vitro and in vivo efficacy data for this compound?
- Methodology : Conduct pharmacokinetic studies to evaluate bioavailability and metabolic stability. For instance, low oral bioavailability (<20%) due to poor solubility may explain reduced in vivo activity. Formulation adjustments (e.g., nanoemulsions) or prodrug strategies (e.g., esterification of the hydroxyethyl group) can improve absorption .
Q. What computational methods are used to predict metabolic pathways and potential toxicity?
- Methodology : Tools like SwissADME and Toxtree predict Phase I/II metabolism (e.g., oxidation of the hydroxyethyl group to a carboxylic acid) and toxicophores. For example, the compound’s thiol derivatives showed hepatotoxicity in silico models, guiding synthetic prioritization .
Q. Data Analysis and Contradictions
Q. How can conflicting results in biological activity between structural analogs be systematically addressed?
- Methodology : Compare analogs using a standardized assay panel. For example, this compound showed 10-fold higher kinase inhibition than its 5-methyl counterpart, attributed to steric hindrance in the active site . Statistical tools (e.g., ANOVA) validate significance, while isothermal titration calorimetry (ITC) quantifies binding affinities .
Q. What experimental designs mitigate batch-to-batch variability in synthetic yields?
Properties
CAS No. |
89711-99-9 |
---|---|
Molecular Formula |
C6H9N3O2 |
Molecular Weight |
155.15 g/mol |
IUPAC Name |
6-amino-5-(2-hydroxyethyl)-1H-pyrimidin-2-one |
InChI |
InChI=1S/C6H9N3O2/c7-5-4(1-2-10)3-8-6(11)9-5/h3,10H,1-2H2,(H3,7,8,9,11) |
InChI Key |
NOYYUQCJSFZZIM-UHFFFAOYSA-N |
Canonical SMILES |
C1=NC(=O)NC(=C1CCO)N |
Origin of Product |
United States |
Disclaimer and Information on In-Vitro Research Products
Please be aware that all articles and product information presented on BenchChem are intended solely for informational purposes. The products available for purchase on BenchChem are specifically designed for in-vitro studies, which are conducted outside of living organisms. In-vitro studies, derived from the Latin term "in glass," involve experiments performed in controlled laboratory settings using cells or tissues. It is important to note that these products are not categorized as medicines or drugs, and they have not received approval from the FDA for the prevention, treatment, or cure of any medical condition, ailment, or disease. We must emphasize that any form of bodily introduction of these products into humans or animals is strictly prohibited by law. It is essential to adhere to these guidelines to ensure compliance with legal and ethical standards in research and experimentation.