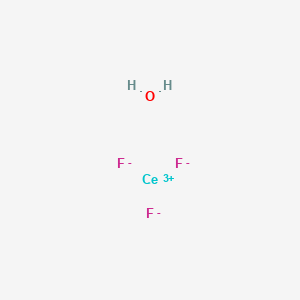
Cerium(III) fluoride xhydrate
- Click on QUICK INQUIRY to receive a quote from our team of experts.
- With the quality product at a COMPETITIVE price, you can focus more on your research.
Overview
Description
It appears as a mineral in the form of fluocerite-(Ce), which is a very rare mineral species related mainly to pegmatites and rarely to oxidation zones of some polymetallic ore deposits . Cerium(III) fluoride is known for its applications in various fields due to its unique properties.
Preparation Methods
Synthetic Routes and Reaction Conditions
Cerium(III) fluoride can be synthesized through several methods. One common method involves the reaction of cerium(III) oxide with hydrofluoric acid. The reaction is typically carried out under controlled conditions to ensure the formation of the desired product:
Ce2O3+6HF→2CeF3+3H2O
Another method involves the direct fluorination of cerium metal using fluorine gas at elevated temperatures. This method requires careful handling due to the reactivity of fluorine gas:
2Ce+3F2→2CeF3
Industrial Production Methods
Industrial production of cerium(III) fluoride often involves the use of cerium(III) chloride as a precursor. The cerium(III) chloride is reacted with hydrofluoric acid to produce cerium(III) fluoride:
CeCl3+3HF→CeF3+3HCl
This method is preferred in industrial settings due to the availability of cerium(III) chloride and the relatively mild reaction conditions.
Chemical Reactions Analysis
Types of Reactions
Cerium(III) fluoride undergoes various types of chemical reactions, including:
Oxidation: Cerium(III) fluoride can be oxidized to cerium(IV) fluoride using strong oxidizing agents such as fluorine gas.
Reduction: Cerium(III) fluoride can be reduced to cerium metal using strong reducing agents.
Substitution: Cerium(III) fluoride can undergo substitution reactions with other halides to form different cerium halides.
Common Reagents and Conditions
Oxidation: Fluorine gas at elevated temperatures.
Reduction: Strong reducing agents such as lithium aluminum hydride.
Substitution: Other halides such as chlorine or bromine in the presence of a suitable solvent.
Major Products Formed
Oxidation: Cerium(IV) fluoride.
Reduction: Cerium metal.
Substitution: Cerium chloride or cerium bromide.
Scientific Research Applications
Cerium(III) fluoride has a wide range of scientific research applications:
Mechanism of Action
The mechanism by which cerium(III) fluoride exerts its effects is primarily related to its ability to undergo redox reactions. Cerium can exist in multiple oxidation states, and the redox cycling between cerium(III) and cerium(IV) plays a crucial role in its chemical behavior. This redox cycling allows cerium(III) fluoride to act as an antioxidant by scavenging reactive oxygen species and preventing oxidative damage .
Comparison with Similar Compounds
Similar Compounds
Lanthanum trifluoride: Similar in structure and properties, but with different applications.
Cerium(IV) fluoride: Higher oxidation state, different reactivity and applications.
Cerium(III) chloride: Different halide, used as a precursor for cerium(III) fluoride synthesis.
Uniqueness
Cerium(III) fluoride is unique due to its specific redox properties and its ability to act as a Faraday rotator material in the visible, near-infrared, and mid-infrared spectral ranges . This makes it particularly valuable in optical applications and in the development of advanced materials for various technologies.
Properties
Molecular Formula |
CeF3H2O |
---|---|
Molecular Weight |
215.127 g/mol |
IUPAC Name |
cerium(3+);trifluoride;hydrate |
InChI |
InChI=1S/Ce.3FH.H2O/h;3*1H;1H2/q+3;;;;/p-3 |
InChI Key |
CRJRBILIOYRLBO-UHFFFAOYSA-K |
Canonical SMILES |
O.[F-].[F-].[F-].[Ce+3] |
Origin of Product |
United States |
Disclaimer and Information on In-Vitro Research Products
Please be aware that all articles and product information presented on BenchChem are intended solely for informational purposes. The products available for purchase on BenchChem are specifically designed for in-vitro studies, which are conducted outside of living organisms. In-vitro studies, derived from the Latin term "in glass," involve experiments performed in controlled laboratory settings using cells or tissues. It is important to note that these products are not categorized as medicines or drugs, and they have not received approval from the FDA for the prevention, treatment, or cure of any medical condition, ailment, or disease. We must emphasize that any form of bodily introduction of these products into humans or animals is strictly prohibited by law. It is essential to adhere to these guidelines to ensure compliance with legal and ethical standards in research and experimentation.