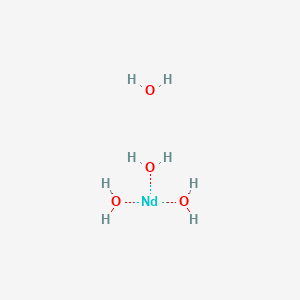
Neodymium(III) hydroxide hydrate
- Click on QUICK INQUIRY to receive a quote from our team of experts.
- With the quality product at a COMPETITIVE price, you can focus more on your research.
Overview
Description
Neodymium(III) hydroxide hydrate is an inorganic compound with the chemical formula Nd(OH)₃•xH₂O. It is a light purple crystalline or powdery substance that is highly insoluble in water. This compound is primarily used in various scientific and industrial applications due to its unique properties and reactivity .
Preparation Methods
Synthetic Routes and Reaction Conditions: Neodymium(III) hydroxide hydrate can be synthesized by reacting neodymium(III) nitrate with ammonia water. The reaction proceeds as follows: [ \text{Nd(NO}_3\text{)}_3 + 3 \text{NH}_3 \cdot \text{H}_2\text{O} \rightarrow \text{Nd(OH)}_3 \downarrow + 3 \text{NH}_4\text{NO}_3 ] In this process, a solution of neodymium(III) nitrate (40g/L) is mixed with ammonia water (0.50 mol/L) at a controlled rate of 1.5 mL/min. Polyethylene glycol is used to maintain the pH, resulting in neodymium(III) hydroxide powder with a grain size of ≤1μm .
Industrial Production Methods: Industrial production of this compound involves similar methods but on a larger scale. The reaction conditions are optimized to ensure high yield and purity. The use of advanced equipment and controlled environments helps in achieving consistent quality and particle size distribution .
Chemical Reactions Analysis
Types of Reactions: Neodymium(III) hydroxide hydrate undergoes various chemical reactions, including:
Acid-Base Reactions: It reacts with acids to form neodymium salts. [ \text{Nd(OH)}_3 + 3 \text{H}^+ \rightarrow \text{Nd}^{3+} + 3 \text{H}_2\text{O} ] For example, with acetic acid: [ \text{Nd(OH)}_3 + 3 \text{CH}_3\text{COOH} \rightarrow \text{Nd(CH}_3\text{COO)}_3 + 3 \text{H}_2\text{O} ]
Common Reagents and Conditions:
Acids: Hydrochloric acid, sulfuric acid, and acetic acid are commonly used.
Conditions: Reactions are typically carried out at room temperature with controlled pH to ensure complete conversion.
Major Products:
Scientific Research Applications
Neodymium(III) hydroxide hydrate has a wide range of applications in scientific research:
Chemistry: Used as a precursor for the synthesis of other neodymium compounds and as a catalyst in various chemical reactions.
Biology: Employed in the study of rare earth elements’ effects on biological systems.
Medicine: Investigated for potential use in medical imaging and targeted drug delivery systems.
Industry: Utilized in the production of optical materials, magnetic materials, and as a component in the extraction of rare earth elements
Mechanism of Action
The mechanism by which neodymium(III) hydroxide hydrate exerts its effects is primarily through its reactivity with acids and its ability to form stable complexes with various ligands. The molecular targets and pathways involved include:
Formation of Neodymium Salts: Reacts with acids to form stable neodymium salts.
Catalytic Activity: Acts as a catalyst in various chemical reactions, facilitating the conversion of reactants to products
Comparison with Similar Compounds
- Praseodymium(III) hydroxide
- Samarium(III) hydroxide
- Lanthanum(III) hydroxide
Comparison: Neodymium(III) hydroxide hydrate is unique due to its specific reactivity and applications. While similar compounds like praseodymium(III) hydroxide and samarium(III) hydroxide share some properties, this compound is particularly valued for its use in optical and magnetic materials. Its ability to form stable complexes and act as a catalyst also sets it apart from other similar compounds .
Properties
Molecular Formula |
H8NdO4 |
---|---|
Molecular Weight |
216.30 g/mol |
IUPAC Name |
neodymium;tetrahydrate |
InChI |
InChI=1S/Nd.4H2O/h;4*1H2 |
InChI Key |
YYOYEZIAOMWDLH-UHFFFAOYSA-N |
Canonical SMILES |
O.O.O.O.[Nd] |
Origin of Product |
United States |
Disclaimer and Information on In-Vitro Research Products
Please be aware that all articles and product information presented on BenchChem are intended solely for informational purposes. The products available for purchase on BenchChem are specifically designed for in-vitro studies, which are conducted outside of living organisms. In-vitro studies, derived from the Latin term "in glass," involve experiments performed in controlled laboratory settings using cells or tissues. It is important to note that these products are not categorized as medicines or drugs, and they have not received approval from the FDA for the prevention, treatment, or cure of any medical condition, ailment, or disease. We must emphasize that any form of bodily introduction of these products into humans or animals is strictly prohibited by law. It is essential to adhere to these guidelines to ensure compliance with legal and ethical standards in research and experimentation.