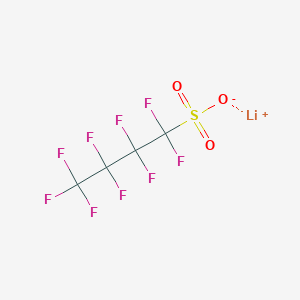
Lithium Nonafluoro-1-butanesulfonate
Overview
Description
Lithium Nonafluoro-1-butanesulfonate is a chemical compound with the molecular formula C4H2F9LiO3S. It is known for its high ionic conductivity and electrochemical stability, making it a valuable component in various applications, particularly in the field of energy storage and electrochemistry .
Mechanism of Action
Target of Action
The primary target of Lithium Nonafluoro-1-butanesulfonate is the cathode-electrolyte interface in sulfonamide electrolytes . It plays a crucial role in stabilizing this interface, thereby enhancing the performance of rechargeable lithium metal batteries (RLMBs) .
Mode of Action
this compound acts as a co-salt and/or electrolyte additive in the solvent . It interacts with its targets by suppressing side reactions occurring at the cathode compartment . This is achieved through the preferential decompositions of the FNFSI− anion .
Biochemical Pathways
The compound affects the electrochemical pathways involved in the operation of RLMBs . By suppressing side reactions at the cathode, it promotes the stability of the cathode-electrolyte interface . This, in turn, enhances the overall performance of the batteries .
Pharmacokinetics
This suggests that it can be readily absorbed and distributed within the electrolyte solution .
Result of Action
The action of this compound results in the stabilization of the cathode-electrolyte interface . This leads to safer and high-performing RLMBs . It also suppresses the growth of lithium dendrites and reduces electrolyte consumption .
Action Environment
The action of this compound is influenced by environmental factors such as temperature and humidity . It is recommended to store the compound under an inert atmosphere at room temperature . It should also be kept away from moisture as it is hygroscopic .
Preparation Methods
Synthetic Routes and Reaction Conditions: Lithium Nonafluoro-1-butanesulfonate can be synthesized by reacting nonafluoro-1-butanesulfonic acid with lithium hydroxide. The reaction typically occurs in an aqueous medium, followed by purification processes to obtain the desired product .
Industrial Production Methods: In industrial settings, the compound is often produced by dissolving nonafluoro-1-butanesulfonic acid in a suitable solvent and then adding lithium hydroxide. The mixture is stirred and heated to facilitate the reaction. After the reaction is complete, the product is purified through crystallization or other separation techniques .
Chemical Reactions Analysis
Types of Reactions: Lithium Nonafluoro-1-butanesulfonate primarily undergoes ionic dissociation in solution, making it an excellent electrolyte. It does not typically participate in oxidation or reduction reactions due to its stability .
Common Reagents and Conditions: The compound is often used in combination with solvents like propylene carbonate and 1,2-dimethoxyethane to form highly conductive electrolytes. These mixtures are prepared under controlled conditions to ensure optimal ionic conductivity .
Major Products Formed: The primary product formed from the dissociation of this compound is the lithium ion (Li+), which is crucial for its role as an electrolyte in lithium-ion batteries .
Scientific Research Applications
Lithium Nonafluoro-1-butanesulfonate has a wide range of applications in scientific research:
Comparison with Similar Compounds
Lithium Trifluoromethanesulfonate: Similar in its use as an electrolyte but has a lower ionic conductivity compared to Lithium Nonafluoro-1-butanesulfonate.
Lithium Bis(trifluoromethanesulfonyl)imide: Known for its high thermal stability and ionic conductivity, making it another popular choice for electrolytes.
Uniqueness: this compound stands out due to its unique combination of high ionic conductivity and electrochemical stability. This makes it particularly suitable for applications requiring long-term stability and high performance, such as in advanced lithium-ion batteries .
Biological Activity
Lithium nonafluoro-1-butanesulfonate (LiNfO) is a novel lithium salt that has garnered attention for its potential applications in lithium-ion batteries (LIBs) due to its unique electrochemical properties. This article explores the biological activity of LiNfO, focusing on its ionic conductivity, stability, and potential implications in battery technology.
Overview of this compound
LiNfO is characterized by its weak cation-anion interactions and high ionic conductivity, making it suitable for use as a lithium ion-conducting salt in non-aqueous liquid electrolytes (NALEs). The compound is synthesized from nonafluoro-1-butanesulfonic acid and lithium hydroxide, resulting in a highly soluble salt that exhibits advantageous properties for electrochemical applications.
Ionic Conductivity and Stability
Research indicates that LiNfO demonstrates significant ionic conductivity, with values reaching up to at ambient temperature when used in a binary solvent mixture of propylene carbonate (PC) and 1,2-dimethoxyethane (DME) . The stability of LiNfO-based electrolytes is noteworthy, providing a potential voltage window of approximately 5 V, which is critical for high-performance LIBs.
Table 1: Ionic Conductivity of LiNfO in Various Solvent Ratios
Solvent Ratio (PC:DME) | Ionic Conductivity () |
---|---|
3:7 | |
1:1 | |
7:3 |
Case Studies
Application in Lithium-Ion Batteries
In a study investigating the performance of LiNfO as an electrolyte in LIBs, researchers observed enhanced cycle life and rate capability compared to traditional lithium salts like LiPF6. The cells demonstrated good reversibility and capacity retention over multiple cycles . For instance, a cell using LiNfO maintained a discharge capacity of 143 mAh/g after 90 cycles at a rate of 0.2 C, showcasing its potential for commercial applications.
Figure 1: Discharge Capacity Over Cycles
Discharge Capacity Graph
Graph illustrating the discharge capacity of Li/NALE/LiCoO2 cells over 90 cycles.
Properties
IUPAC Name |
lithium;1,1,2,2,3,3,4,4,4-nonafluorobutane-1-sulfonate | |
---|---|---|
Source | PubChem | |
URL | https://pubchem.ncbi.nlm.nih.gov | |
Description | Data deposited in or computed by PubChem | |
InChI |
InChI=1S/C4HF9O3S.Li/c5-1(6,3(9,10)11)2(7,8)4(12,13)17(14,15)16;/h(H,14,15,16);/q;+1/p-1 | |
Source | PubChem | |
URL | https://pubchem.ncbi.nlm.nih.gov | |
Description | Data deposited in or computed by PubChem | |
InChI Key |
FEDFHMISXKDOJI-UHFFFAOYSA-M | |
Source | PubChem | |
URL | https://pubchem.ncbi.nlm.nih.gov | |
Description | Data deposited in or computed by PubChem | |
Canonical SMILES |
[Li+].C(C(C(F)(F)S(=O)(=O)[O-])(F)F)(C(F)(F)F)(F)F | |
Source | PubChem | |
URL | https://pubchem.ncbi.nlm.nih.gov | |
Description | Data deposited in or computed by PubChem | |
Molecular Formula |
C4F9LiO3S | |
Source | PubChem | |
URL | https://pubchem.ncbi.nlm.nih.gov | |
Description | Data deposited in or computed by PubChem | |
DSSTOX Substance ID |
DTXSID30635586 | |
Record name | Lithium perfluorobutanesulfonate | |
Source | EPA DSSTox | |
URL | https://comptox.epa.gov/dashboard/DTXSID30635586 | |
Description | DSSTox provides a high quality public chemistry resource for supporting improved predictive toxicology. | |
Molecular Weight |
306.1 g/mol | |
Source | PubChem | |
URL | https://pubchem.ncbi.nlm.nih.gov | |
Description | Data deposited in or computed by PubChem | |
CAS No. |
131651-65-5 | |
Record name | Lithium perfluorobutanesulfonate | |
Source | EPA DSSTox | |
URL | https://comptox.epa.gov/dashboard/DTXSID30635586 | |
Description | DSSTox provides a high quality public chemistry resource for supporting improved predictive toxicology. | |
Record name | lithium perfluorobutanesulfonate | |
Source | European Chemicals Agency (ECHA) | |
URL | https://echa.europa.eu/information-on-chemicals | |
Description | The European Chemicals Agency (ECHA) is an agency of the European Union which is the driving force among regulatory authorities in implementing the EU's groundbreaking chemicals legislation for the benefit of human health and the environment as well as for innovation and competitiveness. | |
Explanation | Use of the information, documents and data from the ECHA website is subject to the terms and conditions of this Legal Notice, and subject to other binding limitations provided for under applicable law, the information, documents and data made available on the ECHA website may be reproduced, distributed and/or used, totally or in part, for non-commercial purposes provided that ECHA is acknowledged as the source: "Source: European Chemicals Agency, http://echa.europa.eu/". Such acknowledgement must be included in each copy of the material. ECHA permits and encourages organisations and individuals to create links to the ECHA website under the following cumulative conditions: Links can only be made to webpages that provide a link to the Legal Notice page. | |
Retrosynthesis Analysis
AI-Powered Synthesis Planning: Our tool employs the Template_relevance Pistachio, Template_relevance Bkms_metabolic, Template_relevance Pistachio_ringbreaker, Template_relevance Reaxys, Template_relevance Reaxys_biocatalysis model, leveraging a vast database of chemical reactions to predict feasible synthetic routes.
One-Step Synthesis Focus: Specifically designed for one-step synthesis, it provides concise and direct routes for your target compounds, streamlining the synthesis process.
Accurate Predictions: Utilizing the extensive PISTACHIO, BKMS_METABOLIC, PISTACHIO_RINGBREAKER, REAXYS, REAXYS_BIOCATALYSIS database, our tool offers high-accuracy predictions, reflecting the latest in chemical research and data.
Strategy Settings
Precursor scoring | Relevance Heuristic |
---|---|
Min. plausibility | 0.01 |
Model | Template_relevance |
Template Set | Pistachio/Bkms_metabolic/Pistachio_ringbreaker/Reaxys/Reaxys_biocatalysis |
Top-N result to add to graph | 6 |
Feasible Synthetic Routes
Disclaimer and Information on In-Vitro Research Products
Please be aware that all articles and product information presented on BenchChem are intended solely for informational purposes. The products available for purchase on BenchChem are specifically designed for in-vitro studies, which are conducted outside of living organisms. In-vitro studies, derived from the Latin term "in glass," involve experiments performed in controlled laboratory settings using cells or tissues. It is important to note that these products are not categorized as medicines or drugs, and they have not received approval from the FDA for the prevention, treatment, or cure of any medical condition, ailment, or disease. We must emphasize that any form of bodily introduction of these products into humans or animals is strictly prohibited by law. It is essential to adhere to these guidelines to ensure compliance with legal and ethical standards in research and experimentation.