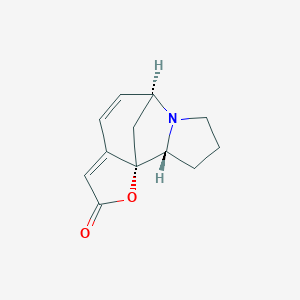
Norsecurinine
Overview
Description
Norsecurinine is a naturally occurring alkaloid belonging to the Securinega family of compounds. This family of natural products has intrigued chemists since the isolation of securinine in 1956. This compound features a pyrrolidine core, distinguishing it from its piperidine-based relatives. It is derived from the plant Flueggea suffruticosa, which is abundant across the Korean peninsula .
Mechanism of Action
Target of Action
Norsecurinine, also known as Ent-norsecurinine, primarily targets α-Tubulin . Tubulin is a globular protein and the main constituent of microtubules, which are critical for maintaining cell structure and function. This compound’s interaction with tubulin can influence cell division and growth, making it a potential candidate for anticancer therapies .
Mode of Action
This compound interacts with α-Tubulin by binding to its large drug-binding cavity . This interaction inhibits microtubule assembly, disrupting the normal function of the cell’s cytoskeleton . The binding capacity of this compound to tubulin varies depending on the alkaloid’s size and structure .
Biochemical Pathways
It’s known that the compound’s interaction with tubulin can disrupt the normal function of the cell’s cytoskeleton . This disruption can lead to changes in cell division and growth, potentially leading to cell death .
Pharmacokinetics
Its ability to bind easily to the large drug-binding cavity on α-tubulin suggests that it may have good bioavailability .
Result of Action
The binding of this compound to α-Tubulin and the subsequent inhibition of microtubule assembly can lead to cell cycle arrest and apoptosis . This makes this compound a potential candidate for anticancer therapies .
Biochemical Analysis
Biochemical Properties
Norsecurinine has been found to interact with α-tubulin, a key protein in the formation of microtubules . The interaction between this compound and α-tubulin has been studied using molecular docking, revealing that this compound can form stable complexes with α/β-tubulin . This interaction is believed to be crucial for the compound’s biological activity .
Cellular Effects
This compound has been shown to have a wide range of effects on cells. It has been characterized as a powerful stimulant of the central nervous system . Furthermore, it has been shown to induce apoptosis via the activation of mitogen-activated protein kinase (MAPK) and other molecular mechanisms . It also has the ability to modulate the PI3K/AKT/mTOR signaling pathways .
Molecular Mechanism
At the molecular level, this compound exerts its effects through various mechanisms. It has been shown to bind to tubulin and inhibit microtubule assembly . This binding interaction is believed to be crucial for its biological activity . Furthermore, this compound has been shown to induce apoptosis via the activation of mitogen-activated protein kinase (MAPK) and other molecular mechanisms .
Metabolic Pathways
It is known that the pyrrolidine moiety in this compound originates from ornithine, marking an early biogenetic divergence .
Preparation Methods
Synthetic Routes and Reaction Conditions: Norsecurinine can be synthesized through a molecular editing strategy that involves the transformation of securinine into this compound. This process includes a single-atom deletion strategy that enables the late-stage conversion of securinane to norsecurinane alkaloids. The key steps involve the transformation of the piperidine-based securinine into the pyrrolidine-based this compound .
Industrial Production Methods: The industrial production of this compound primarily relies on the extraction of securinine from the plant Flueggea suffruticosa, followed by its chemical transformation into this compound. This method leverages the abundant supply of securinine from the plant, making it a viable approach for large-scale production .
Chemical Reactions Analysis
Types of Reactions: Norsecurinine undergoes various chemical reactions, including oxidation, reduction, and substitution reactions. These reactions are facilitated by the presence of functional groups within the molecule that are reactive under specific conditions.
Common Reagents and Conditions:
Oxidation: Common oxidizing agents such as potassium permanganate or chromium trioxide can be used to oxidize this compound.
Reduction: Reducing agents like lithium aluminum hydride or sodium borohydride are employed to reduce this compound.
Substitution: Substitution reactions often involve nucleophiles such as halides or amines under basic or acidic conditions.
Major Products: The major products formed from these reactions depend on the specific reagents and conditions used. For example, oxidation may yield oxidized derivatives of this compound, while reduction can produce reduced forms of the compound .
Scientific Research Applications
Norsecurinine has a wide range of scientific research applications, particularly in the fields of chemistry, biology, medicine, and industry.
Chemistry: In chemistry, this compound serves as a valuable intermediate for the synthesis of more complex securinega alkaloids. Its unique structure allows for the exploration of new synthetic pathways and the development of novel compounds .
Biology and Medicine: this compound exhibits significant biological activity, including anticancer properties. It has been shown to interact with α-tubulin, inhibiting microtubule assembly and leading to mitotic arrest and apoptotic cell death. This makes it a promising candidate for the development of new anticancer drugs .
Industry: In the industrial sector, this compound is used in the production of pharmaceuticals and other bioactive compounds. Its ability to serve as a precursor for various derivatives enhances its industrial value .
Comparison with Similar Compounds
Securinine: A piperidine-based alkaloid with significant biological activity.
Allosecurinine: Another piperidine-based alkaloid with a similar core structure to securinine.
Fluevirine A: A dimeric alkaloid that forms more stable complexes with tubulin than norsecurinine.
Flueggenine I: A this compound dimer that also binds effectively to tubulin.
This compound’s unique pyrrolidine core and its ability to form stable complexes with tubulin distinguish it from these similar compounds, making it a valuable compound for further research and development.
Properties
IUPAC Name |
(1S,8S,13R)-2-oxa-9-azatetracyclo[6.5.1.01,5.09,13]tetradeca-4,6-dien-3-one | |
---|---|---|
Source | PubChem | |
URL | https://pubchem.ncbi.nlm.nih.gov | |
Description | Data deposited in or computed by PubChem | |
InChI |
InChI=1S/C12H13NO2/c14-11-6-8-3-4-9-7-12(8,15-11)10-2-1-5-13(9)10/h3-4,6,9-10H,1-2,5,7H2/t9-,10-,12+/m1/s1 | |
Source | PubChem | |
URL | https://pubchem.ncbi.nlm.nih.gov | |
Description | Data deposited in or computed by PubChem | |
InChI Key |
NBGOALXYAZVRPS-FOGDFJRCSA-N | |
Source | PubChem | |
URL | https://pubchem.ncbi.nlm.nih.gov | |
Description | Data deposited in or computed by PubChem | |
Canonical SMILES |
C1CC2C34CC(N2C1)C=CC3=CC(=O)O4 | |
Source | PubChem | |
URL | https://pubchem.ncbi.nlm.nih.gov | |
Description | Data deposited in or computed by PubChem | |
Isomeric SMILES |
C1C[C@@H]2[C@]34C[C@H](N2C1)C=CC3=CC(=O)O4 | |
Source | PubChem | |
URL | https://pubchem.ncbi.nlm.nih.gov | |
Description | Data deposited in or computed by PubChem | |
Molecular Formula |
C12H13NO2 | |
Source | PubChem | |
URL | https://pubchem.ncbi.nlm.nih.gov | |
Description | Data deposited in or computed by PubChem | |
DSSTOX Substance ID |
DTXSID40949368 | |
Record name | Norsecurinine | |
Source | EPA DSSTox | |
URL | https://comptox.epa.gov/dashboard/DTXSID40949368 | |
Description | DSSTox provides a high quality public chemistry resource for supporting improved predictive toxicology. | |
Molecular Weight |
203.24 g/mol | |
Source | PubChem | |
URL | https://pubchem.ncbi.nlm.nih.gov | |
Description | Data deposited in or computed by PubChem | |
CAS No. |
2650-35-3 | |
Record name | Norsecurinine | |
Source | ChemIDplus | |
URL | https://pubchem.ncbi.nlm.nih.gov/substance/?source=chemidplus&sourceid=0002650353 | |
Description | ChemIDplus is a free, web search system that provides access to the structure and nomenclature authority files used for the identification of chemical substances cited in National Library of Medicine (NLM) databases, including the TOXNET system. | |
Record name | Norsecurinine | |
Source | EPA DSSTox | |
URL | https://comptox.epa.gov/dashboard/DTXSID40949368 | |
Description | DSSTox provides a high quality public chemistry resource for supporting improved predictive toxicology. | |
Record name | NORSECURININE | |
Source | FDA Global Substance Registration System (GSRS) | |
URL | https://gsrs.ncats.nih.gov/ginas/app/beta/substances/OPF6A516XH | |
Description | The FDA Global Substance Registration System (GSRS) enables the efficient and accurate exchange of information on what substances are in regulated products. Instead of relying on names, which vary across regulatory domains, countries, and regions, the GSRS knowledge base makes it possible for substances to be defined by standardized, scientific descriptions. | |
Explanation | Unless otherwise noted, the contents of the FDA website (www.fda.gov), both text and graphics, are not copyrighted. They are in the public domain and may be republished, reprinted and otherwise used freely by anyone without the need to obtain permission from FDA. Credit to the U.S. Food and Drug Administration as the source is appreciated but not required. | |
Retrosynthesis Analysis
AI-Powered Synthesis Planning: Our tool employs the Template_relevance Pistachio, Template_relevance Bkms_metabolic, Template_relevance Pistachio_ringbreaker, Template_relevance Reaxys, Template_relevance Reaxys_biocatalysis model, leveraging a vast database of chemical reactions to predict feasible synthetic routes.
One-Step Synthesis Focus: Specifically designed for one-step synthesis, it provides concise and direct routes for your target compounds, streamlining the synthesis process.
Accurate Predictions: Utilizing the extensive PISTACHIO, BKMS_METABOLIC, PISTACHIO_RINGBREAKER, REAXYS, REAXYS_BIOCATALYSIS database, our tool offers high-accuracy predictions, reflecting the latest in chemical research and data.
Strategy Settings
Precursor scoring | Relevance Heuristic |
---|---|
Min. plausibility | 0.01 |
Model | Template_relevance |
Template Set | Pistachio/Bkms_metabolic/Pistachio_ringbreaker/Reaxys/Reaxys_biocatalysis |
Top-N result to add to graph | 6 |
Feasible Synthetic Routes
A: Norsecurinine has been identified as a potential α-tubulin binding agent. [, ] Molecular docking studies suggest that this compound-type alkaloids, particularly dimeric forms like flueggine I, can bind to the pironetin site of α-tubulin, interacting with regions near the colchicine-binding area and cysteine-316 residue. [] While the exact mechanism of action requires further investigation, this binding to tubulin likely disrupts microtubule assembly, similar to other known tubulin-binding agents. [] This disruption can have significant downstream effects on cellular processes like mitosis, intracellular transport, and cell morphology, potentially contributing to the observed anticancer activity of this compound and related compounds. [, ]
ANone: While the provided abstracts do not contain detailed spectroscopic data for this compound, we can provide the following information:
- Molecular Formula: C13H15NO2 []
- Structure: this compound possesses a tetracyclic framework characteristic of Securinega alkaloids, featuring a pyrrolidine core instead of the piperidine found in the related securinine. [, , ] The structure also includes a conjugated enone system within one of the rings. [, ]
ANone: The provided abstracts do not offer specific details regarding the material compatibility and stability of this compound under various conditions. This information would require further investigation and dedicated studies.
ANone: The provided research primarily focuses on the isolation, synthesis, and biological activity of this compound and its analogues. There is no mention of this compound exhibiting catalytic properties or being employed in catalytic applications.
A: Research suggests that the dimeric nature of this compound-based alkaloids can influence their interaction with α-tubulin. [, ] Dimeric alkaloids like flueggine I demonstrate potentially enhanced binding compared to monomeric counterparts, suggesting that increasing the size and structural complexity could improve tubulin binding. [] Additionally, the presence of the indole moiety, as seen in flueggenine E, also appears to contribute to stronger tubulin interactions. []
ANone: The provided abstracts do not contain detailed information regarding the stability of this compound under various conditions or specific formulation strategies employed to enhance its properties. Further investigation is needed to explore these aspects.
ANone: The historical context of this compound research is rooted in the investigation of Securinega alkaloids:
- Early Isolation: this compound was first isolated from the roots of Securinega virosa in the mid-20th century, along with hordenine. []
- Structural Elucidation: The structure and absolute stereochemistry of this compound were subsequently determined, establishing its relationship to other Securinega alkaloids. [, , ]
- Synthetic Efforts: Numerous total syntheses of this compound have been achieved over the years, highlighting its significance as a synthetic target and contributing to the understanding of its chemistry. [, , , , , , , , , , , , , , , , , , , ]
- Biological Activity Exploration: More recent research has focused on exploring the biological activities of this compound and related alkaloids, particularly their potential as anticancer agents due to their interaction with tubulin. [, , ]
ANone: this compound research showcases significant cross-disciplinary applications and synergies, primarily bridging:
- Organic Chemistry: Numerous total syntheses highlight the intricate challenges and advancements in synthetic organic chemistry related to constructing this compound's complex tetracyclic framework. [, , , , , ]
- Medicinal Chemistry: The exploration of this compound's biological activity, particularly its anti-cancer potential and interaction with tubulin, demonstrates a clear link to medicinal chemistry and drug discovery efforts. [, , ]
- Computational Chemistry: Molecular docking studies applied to understand this compound's binding to α-tubulin exemplify the growing role of computational approaches in complementing experimental research in drug discovery. [, ]
- Natural Product Chemistry: this compound's origin as a natural product from Securinega virosa underscores the importance of natural product isolation, characterization, and investigation for discovering new bioactive compounds. [, , , ]
Disclaimer and Information on In-Vitro Research Products
Please be aware that all articles and product information presented on BenchChem are intended solely for informational purposes. The products available for purchase on BenchChem are specifically designed for in-vitro studies, which are conducted outside of living organisms. In-vitro studies, derived from the Latin term "in glass," involve experiments performed in controlled laboratory settings using cells or tissues. It is important to note that these products are not categorized as medicines or drugs, and they have not received approval from the FDA for the prevention, treatment, or cure of any medical condition, ailment, or disease. We must emphasize that any form of bodily introduction of these products into humans or animals is strictly prohibited by law. It is essential to adhere to these guidelines to ensure compliance with legal and ethical standards in research and experimentation.