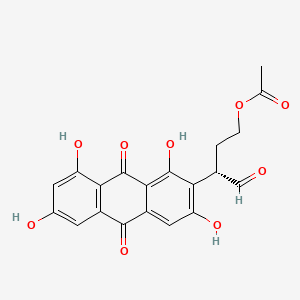
Versiconal acetate
- Click on QUICK INQUIRY to receive a quote from our team of experts.
- With the quality product at a COMPETITIVE price, you can focus more on your research.
Overview
Description
Versiconal acetate is a metabolite produced by the fungus Aspergillus parasiticus. It plays a crucial role in the biosynthesis of aflatoxins, which are highly toxic and carcinogenic compounds. This compound is an intermediate in the aflatoxin biosynthetic pathway and is essential for the conversion of precursor molecules into aflatoxins .
Preparation Methods
Synthetic Routes and Reaction Conditions: Versiconal acetate is typically synthesized through the metabolic processes of Aspergillus parasiticus. The biosynthesis involves the conversion of 1-hydroxyversicolorone to versiconal hemiacetal acetate, which is then converted to this compound . The key enzymes involved in this process include Baeyer-Villiger monooxygenase and esterase .
Industrial Production Methods: Industrial production of this compound is not common due to its specific role in aflatoxin biosynthesis. it can be produced in laboratory settings by culturing Aspergillus parasiticus under controlled conditions and extracting the compound from the fungal cultures .
Chemical Reactions Analysis
Types of Reactions: Versiconal acetate undergoes several types of chemical reactions, including oxidation, reduction, and hydrolysis. The primary reactions involve the conversion of this compound to versiconal and versiconol acetate to versiconol .
Common Reagents and Conditions:
Major Products: The major products formed from these reactions include versiconal and versiconol, which are further intermediates in the aflatoxin biosynthetic pathway .
Scientific Research Applications
Versiconal acetate has several scientific research applications, particularly in the study of aflatoxin biosynthesis. It is used to understand the enzymatic steps and genetic regulation involved in the production of aflatoxins . Additionally, it serves as a model compound for studying the metabolic pathways of polyketide-derived furanocoumarins .
In the field of biotechnology, this compound is used to investigate the role of specific enzymes and genes in fungal metabolism.
Mechanism of Action
Versiconal acetate exerts its effects through its role in the aflatoxin biosynthetic pathway. The compound is converted to versiconal by esterase enzymes, which then undergoes further transformations to produce aflatoxins . The key molecular targets and pathways involved include the Baeyer-Villiger monooxygenase and esterase enzymes that catalyze the conversion of precursor molecules to aflatoxins .
Comparison with Similar Compounds
Versiconal acetate is similar to other intermediates in the aflatoxin biosynthetic pathway, such as versiconal hemiacetal acetate and versiconol acetate . it is unique in its specific role in the conversion of precursor molecules to aflatoxins. Other similar compounds include:
Versiconal hemiacetal acetate: An intermediate that is converted to this compound by esterase enzymes.
Versiconol acetate: Another intermediate that is converted to versiconol by esterase enzymes.
This compound’s uniqueness lies in its specific function within the aflatoxin biosynthetic pathway and its role in the production of highly toxic and carcinogenic aflatoxins .
Biological Activity
Versiconal acetate (VOAc) is a significant compound in the biosynthesis of aflatoxins, potent carcinogenic metabolites produced by certain fungi, particularly Aspergillus parasiticus and Aspergillus flavus. Understanding the biological activity of VOAc involves exploring its metabolic pathways, enzymatic conversions, and potential implications for health and agriculture.
1. Metabolic Pathways
This compound is part of a complex metabolic grid involved in aflatoxin biosynthesis. The conversion of VOAc to versiconol (VOH) is catalyzed by various esterases. Research indicates that the esterase encoded by the estA gene plays a crucial role in this conversion, alongside other metabolic intermediates such as versiconal hemiacetal acetate (VHA) .
Key Reactions in Aflatoxin Biosynthesis:
- Conversion Steps:
- VOAc → VOH
- VHA → VOH
- Enzymatic Inhibition:
2. Enzymatic Activity and Characterization
The enzymatic activity related to VOAc involves several key enzymes:
- Esterases: Responsible for hydrolyzing esters to produce alcohols and acids, crucial for converting VOAc to VOH.
- Dehydrogenases: Facilitate the oxidation-reduction reactions necessary for the transformation of various intermediates in aflatoxin biosynthesis.
Table 1: Enzymes Involved in the Metabolism of this compound
3. Case Studies and Experimental Findings
A series of experiments have demonstrated the biological activity of VOAc through various methodologies:
- In vitro Studies: Experiments using cell extracts from A. parasiticus mutants showed that conversion rates of VOAc to VOH were significantly reduced when specific esterase genes were knocked out .
- Feeding Experiments: Non-aflatoxigenic mutants were fed with VHA and VOAc, resulting in new aflatoxin production, showcasing the potential for these compounds to contribute to fungal toxicity under certain conditions .
4. Health Implications
The biological activity of this compound has direct implications for food safety and public health due to its role in aflatoxin production:
- Carcinogenicity: Aflatoxins are recognized as potent carcinogens, and understanding the pathways involving VOAc can help mitigate risks associated with contaminated food products.
- Agricultural Impact: The presence of VOAc and its metabolic products can influence crop health and safety, necessitating further research into pest control measures that target these metabolic pathways.
Properties
CAS No. |
52021-61-1 |
---|---|
Molecular Formula |
C20H16O9 |
Molecular Weight |
400.3 g/mol |
IUPAC Name |
[(3S)-4-oxo-3-(1,3,6,8-tetrahydroxy-9,10-dioxoanthracen-2-yl)butyl] acetate |
InChI |
InChI=1S/C20H16O9/c1-8(22)29-3-2-9(7-21)15-14(25)6-12-17(19(15)27)20(28)16-11(18(12)26)4-10(23)5-13(16)24/h4-7,9,23-25,27H,2-3H2,1H3/t9-/m1/s1 |
InChI Key |
YTGNIIJMSNZCOG-SECBINFHSA-N |
Isomeric SMILES |
CC(=O)OCC[C@H](C=O)C1=C(C=C2C(=C1O)C(=O)C3=C(C2=O)C=C(C=C3O)O)O |
Canonical SMILES |
CC(=O)OCCC(C=O)C1=C(C=C2C(=C1O)C(=O)C3=C(C2=O)C=C(C=C3O)O)O |
Origin of Product |
United States |
Disclaimer and Information on In-Vitro Research Products
Please be aware that all articles and product information presented on BenchChem are intended solely for informational purposes. The products available for purchase on BenchChem are specifically designed for in-vitro studies, which are conducted outside of living organisms. In-vitro studies, derived from the Latin term "in glass," involve experiments performed in controlled laboratory settings using cells or tissues. It is important to note that these products are not categorized as medicines or drugs, and they have not received approval from the FDA for the prevention, treatment, or cure of any medical condition, ailment, or disease. We must emphasize that any form of bodily introduction of these products into humans or animals is strictly prohibited by law. It is essential to adhere to these guidelines to ensure compliance with legal and ethical standards in research and experimentation.