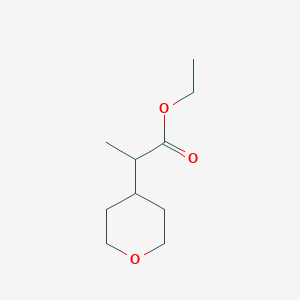
Ethyl 2-(oxan-4-yl)propanoate
Overview
Description
Ethyl 2-(oxan-4-yl)propanoate is a chemical compound with the CAS Number: 920297-27-4 . It has a molecular weight of 186.25 and its IUPAC name is ethyl 2-tetrahydro-2H-pyran-4-ylpropanoate . It is a liquid at room temperature .
Molecular Structure Analysis
The InChI code for Ethyl 2-(oxan-4-yl)propanoate is 1S/C10H18O3/c1-3-13-10(11)8(2)9-4-6-12-7-5-9/h8-9H,3-7H2,1-2H3 . This code provides a specific representation of the molecule’s structure.Physical And Chemical Properties Analysis
Ethyl 2-(oxan-4-yl)propanoate is a liquid at room temperature . The compound’s other physical and chemical properties such as boiling point, melting point, and solubility are not specified in the retrieved data.Scientific Research Applications
Chemical Synthesis and Characterization
- Ethyl 2-oxo-3-(4-oxo-4Н-chromen-2-yl)propanoates have been studied for their reactivity with S-methylisothiosemicarbazide hydroiodide, resulting in the formation of various compounds with potential applications in chemical synthesis (Vetyugova et al., 2018). The same study was repeated by different authors emphasizing the compound's significance in chemical reactions (Сосновских et al., 2018).
Spectroscopic Studies
- Spectroscopic and diffractometric techniques have been employed to characterize polymorphic forms of a compound structurally related to Ethyl 2-(oxan-4-yl)propanoate, highlighting the compound's role in investigational pharmaceutical applications (Vogt et al., 2013).
Chemical Synthesis of Polyheterocyclic Compounds
- Ethyl 2-(furan-2-yl)-3-oxo-3-(aryl-2-yl)propanoates were involved in the photoinduced direct oxidative annulation, leading to the synthesis of highly functionalized polyheterocyclic compounds, demonstrating the compound's utility in creating complex chemical structures (Zhang et al., 2017).
Chiral Epoxide Synthesis
- Ethyl and methyl (S)-3-(oxiran-2-yl)propanoates, closely related to Ethyl 2-(oxan-4-yl)propanoate, were synthesized from levoglucosenone, demonstrating an innovative chemo-enzymatic pathway for producing valuable precursors in chemical syntheses (Peru et al., 2016).
Coordination Chemistry
- The synthesis and structural determination of Ethyl 2,2-bis{[(benzoylcarbamothioyl)oxy]methyl}propanoate and its complexes with copper(II) and cobalt(II) ions were explored, indicating its significance in coordination chemistry and material science (Maksimov et al., 2020).
Crystallography and Solid-Liquid Equilibrium
- Studies involving the crystallization of Nafronyl oxalate, a compound structurally related to Ethyl 2-(oxan-4-yl)propanoate, highlighted its use in understanding the solid-liquid equilibrium and crystal packing, which is crucial for drug formulation and material science (Olbrycht et al., 2016).
Safety and Hazards
The safety information for Ethyl 2-(oxan-4-yl)propanoate indicates that it is a potential hazard. The compound has been assigned the GHS07 pictogram and the signal word "Warning" . Hazard statements include H315, H319, and H335 , which correspond to skin irritation, eye irritation, and respiratory irritation, respectively. Precautionary measures include avoiding breathing the mist/vapors/spray and avoiding contact with skin and eyes .
Mechanism of Action
Mode of Action
The compound’s interaction with its targets and the resulting changes at the molecular and cellular level are areas of ongoing research .
Pharmacokinetics
These properties play a crucial role in determining the bioavailability of the compound, which is a key factor in its pharmacological efficacy .
Result of Action
Understanding these effects is crucial for predicting the compound’s potential therapeutic applications .
Action Environment
Factors such as temperature, pH, and the presence of other compounds can potentially affect the compound’s action .
properties
IUPAC Name |
ethyl 2-(oxan-4-yl)propanoate | |
---|---|---|
Source | PubChem | |
URL | https://pubchem.ncbi.nlm.nih.gov | |
Description | Data deposited in or computed by PubChem | |
InChI |
InChI=1S/C10H18O3/c1-3-13-10(11)8(2)9-4-6-12-7-5-9/h8-9H,3-7H2,1-2H3 | |
Source | PubChem | |
URL | https://pubchem.ncbi.nlm.nih.gov | |
Description | Data deposited in or computed by PubChem | |
InChI Key |
BZQJYCUZVIJNJJ-UHFFFAOYSA-N | |
Source | PubChem | |
URL | https://pubchem.ncbi.nlm.nih.gov | |
Description | Data deposited in or computed by PubChem | |
Canonical SMILES |
CCOC(=O)C(C)C1CCOCC1 | |
Source | PubChem | |
URL | https://pubchem.ncbi.nlm.nih.gov | |
Description | Data deposited in or computed by PubChem | |
Molecular Formula |
C10H18O3 | |
Source | PubChem | |
URL | https://pubchem.ncbi.nlm.nih.gov | |
Description | Data deposited in or computed by PubChem | |
Molecular Weight |
186.25 g/mol | |
Source | PubChem | |
URL | https://pubchem.ncbi.nlm.nih.gov | |
Description | Data deposited in or computed by PubChem | |
CAS RN |
920297-27-4 | |
Record name | ethyl 2-(oxan-4-yl)propanoate | |
Source | European Chemicals Agency (ECHA) | |
URL | https://echa.europa.eu/information-on-chemicals | |
Description | The European Chemicals Agency (ECHA) is an agency of the European Union which is the driving force among regulatory authorities in implementing the EU's groundbreaking chemicals legislation for the benefit of human health and the environment as well as for innovation and competitiveness. | |
Explanation | Use of the information, documents and data from the ECHA website is subject to the terms and conditions of this Legal Notice, and subject to other binding limitations provided for under applicable law, the information, documents and data made available on the ECHA website may be reproduced, distributed and/or used, totally or in part, for non-commercial purposes provided that ECHA is acknowledged as the source: "Source: European Chemicals Agency, http://echa.europa.eu/". Such acknowledgement must be included in each copy of the material. ECHA permits and encourages organisations and individuals to create links to the ECHA website under the following cumulative conditions: Links can only be made to webpages that provide a link to the Legal Notice page. | |
Synthesis routes and methods I
Procedure details
Synthesis routes and methods II
Procedure details
Retrosynthesis Analysis
AI-Powered Synthesis Planning: Our tool employs the Template_relevance Pistachio, Template_relevance Bkms_metabolic, Template_relevance Pistachio_ringbreaker, Template_relevance Reaxys, Template_relevance Reaxys_biocatalysis model, leveraging a vast database of chemical reactions to predict feasible synthetic routes.
One-Step Synthesis Focus: Specifically designed for one-step synthesis, it provides concise and direct routes for your target compounds, streamlining the synthesis process.
Accurate Predictions: Utilizing the extensive PISTACHIO, BKMS_METABOLIC, PISTACHIO_RINGBREAKER, REAXYS, REAXYS_BIOCATALYSIS database, our tool offers high-accuracy predictions, reflecting the latest in chemical research and data.
Strategy Settings
Precursor scoring | Relevance Heuristic |
---|---|
Min. plausibility | 0.01 |
Model | Template_relevance |
Template Set | Pistachio/Bkms_metabolic/Pistachio_ringbreaker/Reaxys/Reaxys_biocatalysis |
Top-N result to add to graph | 6 |
Feasible Synthetic Routes
Disclaimer and Information on In-Vitro Research Products
Please be aware that all articles and product information presented on BenchChem are intended solely for informational purposes. The products available for purchase on BenchChem are specifically designed for in-vitro studies, which are conducted outside of living organisms. In-vitro studies, derived from the Latin term "in glass," involve experiments performed in controlled laboratory settings using cells or tissues. It is important to note that these products are not categorized as medicines or drugs, and they have not received approval from the FDA for the prevention, treatment, or cure of any medical condition, ailment, or disease. We must emphasize that any form of bodily introduction of these products into humans or animals is strictly prohibited by law. It is essential to adhere to these guidelines to ensure compliance with legal and ethical standards in research and experimentation.