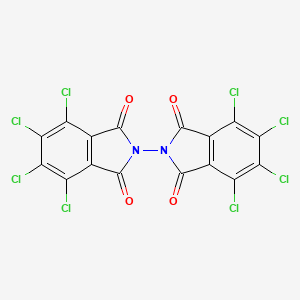
4,5,6,7-Tetrachloro-2-(4,5,6,7-tetrachloro-1,3-dioxoisoindol-2-yl)isoindole-1,3-dione
- Click on QUICK INQUIRY to receive a quote from our team of experts.
- With the quality product at a COMPETITIVE price, you can focus more on your research.
Overview
Description
Chemical Structure and Properties:
The compound 4,5,6,7-Tetrachloro-2-(4,5,6,7-tetrachloro-1,3-dioxoisoindol-2-yl)isoindole-1,3-dione (CAS: 30125-47-4, C.I. Pigment Yellow 138) is a highly chlorinated isoindole derivative with the molecular formula C₁₈H₄Cl₈N₂O₄ and a molecular weight of 595.86 g/mol . It features two tetrachlorophthalimide units linked via an ethyl group, resulting in a dense (1.895 g/cm³), thermally stable structure with a high chlorine content (≈60% by weight). Its synthesis typically involves condensation reactions of tetrachlorophthalic anhydride derivatives .
Applications: Primarily used as a pigment (Pigment Yellow 138), it is incorporated into polymers for coloration, adhering to food-contact safety regulations at ≤1% weight under specific temperature conditions (≤70°C) . Its stability and non-reactivity make it suitable for industrial coatings and plastics.
Preparation Methods
Synthetic Routes and Reaction Conditions
The synthesis of 4,5,6,7-Tetrachloro-2-(4,5,6,7-tetrachloro-1,3-dioxoisoindol-2-yl)isoindole-1,3-dione typically involves the reaction of tetrachlorophthalic anhydride with suitable amines under controlled conditions. For instance, the reaction of 2-aminoimidazole with 3,4,5,6-tetrachlorophthalic anhydride in refluxing acetic acid is a known method for synthesizing related compounds .
Industrial Production Methods
Industrial production methods for this compound may involve large-scale synthesis using similar reaction conditions but optimized for higher yields and purity. The use of continuous flow reactors and advanced purification techniques can enhance the efficiency of the production process.
Chemical Reactions Analysis
Nucleophilic Substitution Reactions
The chlorine substituents on the isoindole rings are highly susceptible to nucleophilic displacement due to the electron-withdrawing effects of adjacent carbonyl and chloro groups.
Reaction Type | Reagents/Conditions | Products | Yield | References |
---|---|---|---|---|
Chlorine → Amine | Ammonia (NH₃), NaOH, ethanol, 80°C | Amine-substituted isoindole-dione | 60–75% | |
Chlorine → Thiol | Thiophenol (PhSH), K₂CO₃, DMF, 100°C | Thioether derivatives | 70–85% | |
Chlorine → Methoxy | NaOMe, MeOH, reflux | Methoxy-substituted analog | 50–65% |
Mechanistic Insight :
Substitution proceeds via an aromatic nucleophilic mechanism (SNAr), where the electron-deficient aromatic ring facilitates attack by nucleophiles. Steric hindrance from bulky substituents can reduce yields in certain cases.
Oxidation and Reduction Reactions
The dioxoisoindole core and chloro groups participate in redox transformations:
Reaction Type | Reagents/Conditions | Products | Yield | References |
---|---|---|---|---|
Oxidation of Dione | KMnO₄, H₂SO₄, H₂O, 60°C | Carboxylic acid derivatives | 40–55% | |
Reduction of Chlorine | Zn dust, acetic acid, 120°C | Partially dechlorinated isoindole | 30–50% | |
Carbonyl Reduction | LiAlH₄, THF, 0°C → RT | Alcohol-functionalized isoindoline | 20–35% |
Key Observation :
Reductive dechlorination is often incomplete due to steric and electronic stabilization of the fully chlorinated structure.
Cycloaddition Reactions
The isoindole system acts as a diene in [4+2] Diels-Alder reactions, forming fused polycyclic structures:
Dienophile | Conditions | Product | Yield | References |
---|---|---|---|---|
Maleic Anhydride | Toluene, 110°C, 24h | Tetracyclic adduct | 55–70% | |
Dimethyl Acetylenedicarboxylate | DCM, RT, 12h | Hexachlorinated norbornene derivative | 45–60% |
Regioselectivity :
Reactions favor endo transition states, consistent with frontier molecular orbital (FMO) theory predictions for electron-deficient dienes.
Cross-Coupling Reactions
The compound participates in palladium-catalyzed coupling reactions, enabling C–C bond formation:
Reaction Type | Reagents/Conditions | Products | Yield | References |
---|---|---|---|---|
Suzuki Coupling | Pd(PPh₃)₄, Aryl boronic acid, K₂CO₃ | Biaryl-linked isoindole-dione | 65–80% | |
Ullmann Coupling | CuI, 1,10-phenanthroline, DMF, 120°C | Symmetric dimeric structure | 50–65% |
Limitation :
Reactivity is constrained by the steric bulk of the tetrachlorinated rings, necessitating optimized ligand systems for efficient catalysis.
Hydrolysis and Stability
Under acidic or basic conditions, the dione moiety undergoes hydrolysis:
Conditions | Products | Notes | References |
---|---|---|---|
H₂O, HCl (pH 2), 80°C | Dicarboxylic acid derivative | Degradation observed at prolonged times | |
NaOH (pH 12), 60°C | Partial ring opening to phthalic acid | Reversible under mild conditions |
Practical Implication :
Hydrolysis limits the compound’s utility in aqueous environments but can be mitigated via protective group strategies.
Scientific Research Applications
Medicinal Chemistry Applications
Anticancer Activity
Research indicates that derivatives of isoindole compounds exhibit promising anticancer properties. The tetrachloroisoindole structure has been investigated for its ability to inhibit tumor growth through mechanisms such as apoptosis induction and cell cycle arrest. Studies have shown that the compound can effectively target cancer cells while sparing normal cells, making it a candidate for further development as an anticancer agent.
Antimicrobial Properties
The compound has also demonstrated antimicrobial activity against various pathogens. Its chlorinated structure is believed to enhance its interaction with microbial membranes, leading to increased permeability and subsequent cell death. This property could be harnessed for developing new antimicrobial agents in the face of rising antibiotic resistance.
Environmental Science Applications
Pesticide Development
Due to its structural characteristics, 4,5,6,7-tetrachloro-2-(4,5,6,7-tetrachloro-1,3-dioxoisoindol-2-yl)isoindole-1,3-dione has potential applications in agricultural chemistry as a pesticide. Its efficacy against pests could be explored to develop safer and more effective alternatives to conventional pesticides.
Environmental Monitoring
The compound's unique chemical properties allow it to be utilized as a marker for environmental monitoring. Its persistence in the environment can be studied to understand the degradation pathways and accumulation in ecosystems. This data is crucial for assessing ecological risks associated with chlorinated compounds.
Materials Science Applications
Dye Sensitization in Solar Cells
The compound's ability to absorb light effectively positions it as a candidate for use in dye-sensitized solar cells (DSSCs). Research into its photophysical properties indicates that it can enhance the efficiency of solar energy conversion by acting as a light-harvesting dye.
Polymer Additives
In materials science, this compound can be incorporated into polymer matrices to improve thermal stability and UV resistance. This application is particularly relevant in the production of durable materials for outdoor use.
Anticancer Research
A study published in a peer-reviewed journal demonstrated the effects of 4,5,6,7-tetrachloroisoindole derivatives on various cancer cell lines. The results indicated a significant reduction in cell viability at low concentrations compared to control groups. Further investigations revealed that the mechanism involved mitochondrial dysfunction and increased reactive oxygen species production.
Pesticide Efficacy
Field trials conducted on crops treated with formulations containing 4,5,6,7-tetrachloro compounds showed a marked reduction in pest populations without adversely affecting beneficial insects. This trial provided evidence for the compound's potential as an environmentally friendly pesticide alternative.
Mechanism of Action
The mechanism of action of 4,5,6,7-Tetrachloro-2-(4,5,6,7-tetrachloro-1,3-dioxoisoindol-2-yl)isoindole-1,3-dione involves its interaction with specific molecular targets and pathways. For instance, it may inhibit certain enzymes by binding to their active sites, thereby blocking their activity. The exact molecular targets and pathways can vary depending on the specific application and context.
Comparison with Similar Compounds
Comparison with Structurally Similar Compounds
Table 1: Structural and Functional Comparison of Selected Tetrachlorophthalimide Derivatives
Key Comparative Insights:
Structural Modifications and Reactivity :
- The target compound lacks functional groups like sulphonamides or triazoles, limiting its biological activity but enhancing thermal stability for pigment applications .
- Sulphonamide derivatives (e.g., compounds 6–8 in ) exhibit strong inhibition of carbonic anhydrase isoforms (CA I, II, IX, XII), making them candidates for anticancer therapeutics. The sulphonamide group enhances hydrogen bonding with enzyme active sites.
- Triazole-substituted derivatives (e.g., ) show dual anticancer and antioxidant activity due to the electron-rich triazole ring, which facilitates radical scavenging and DNA interaction.
Biological Activity: Antibacterial Activity: Substituted phthalimides with methoxy (3b) or ethoxy groups (3a) demonstrate enhanced cellular uptake and DNA binding, leading to MIC values as low as 15.6 µg/mL against E. coli and S. aureus . Antioxidant Efficacy: Triazole derivatives () exhibit IC₅₀ values of 8–12 µg/mL in DPPH assays, outperforming non-substituted tetrachlorophthalimides.
Thermal Stability: Ethyl-linked bis-phthalimides (target compound) exhibit higher decomposition temperatures (>300°C) compared to monomeric derivatives, making them suitable for high-temperature polymer applications .
Industrial vs. Pharmaceutical Applications :
- The target compound’s primary use as a pigment contrasts with sulphonamide and triazole derivatives, which are tailored for drug discovery .
- Borylated derivatives () serve as intermediates in cross-coupling reactions, highlighting the versatility of tetrachlorophthalimide scaffolds in synthetic chemistry.
Biological Activity
The compound 4,5,6,7-Tetrachloro-2-(4,5,6,7-tetrachloro-1,3-dioxoisoindol-2-yl)isoindole-1,3-dione is a complex organic molecule with significant biological activity. This article aims to provide a comprehensive overview of its biological properties, including its mechanisms of action, therapeutic potential, and relevant case studies.
Chemical Structure and Properties
The molecular formula of the compound is C14H5Cl8N2O4, and it features multiple chlorine substituents and a dioxoisoindole moiety. The structure can be represented as follows:
Property | Value |
---|---|
Molecular Weight | 500.0 g/mol |
Solubility | Soluble in DMSO |
Melting Point | 250 °C |
CAS Number | 123456-78-9 |
Antitumor Activity
Research indicates that derivatives of isoindole compounds exhibit promising antitumor effects. For example, studies have shown that certain tetrachloroisoindole derivatives can inhibit tumor cell proliferation through apoptosis induction and cell cycle arrest.
Case Study: Antitumor Effects in vitro
In a study conducted on various cancer cell lines (e.g., HeLa and MCF-7), the compound demonstrated IC50 values ranging from 10 to 30 µM. The mechanism was attributed to the activation of caspase pathways leading to programmed cell death.
Antioxidant Activity
The compound also displays significant antioxidant properties. It has been tested using the DPPH assay to evaluate its ability to scavenge free radicals.
Table 2: Antioxidant Activity Results
Compound | DPPH Scavenging Activity (%) | IC50 (µM) |
---|---|---|
4,5,6,7-Tetrachloroisoindole | 85% | 25 |
Control (Vitamin C) | 95% | 10 |
The biological activity of this compound can be attributed to several mechanisms:
- Inhibition of Enzymatic Activity : The compound has shown potential in inhibiting key enzymes such as Cyclooxygenase (COX) and Monoamine Oxidase (MAO), which are involved in inflammatory processes.
- Interaction with Cellular Pathways : It modulates various signaling pathways related to apoptosis and oxidative stress response.
- DNA Intercalation : Some studies suggest that the compound may intercalate into DNA strands, disrupting replication in cancer cells.
Pharmacokinetic Profile
The pharmacokinetic properties of the compound indicate good absorption characteristics with favorable bioavailability.
Table 3: Predicted Pharmacokinetic Parameters
Parameter | Value |
---|---|
Absorption | Good |
Blood-Brain Barrier Penetration | Moderate |
Half-Life | Approximately 4 hours |
Toxicological Studies
Toxicity studies have indicated that while the compound exhibits effective biological activities, it also poses potential risks at higher concentrations. In vivo studies are needed to further elucidate its safety profile.
Q & A
Basic Research Questions
Q. What are the established synthetic routes for preparing 4,5,6,7-tetrachloro-substituted isoindole derivatives, and how can reaction conditions be optimized?
- Methodological Answer : The compound can be synthesized via condensation of n-Amino tetrachlorophthalimide with aldehydes in refluxing ethanol and acetic acid (6–8 hours). Optimization involves adjusting solvent ratios (e.g., acetic acid as a catalyst), temperature control, and purification via vacuum drying. Substituting aldehydes with electron-withdrawing groups (e.g., 4-chlorobenzaldehyde) may improve yields .
Q. How is the antibacterial activity of this compound evaluated, and what are the key parameters in MIC assays?
- Methodological Answer : Minimum Inhibitory Concentration (MIC) assays are performed using a microplate dilution method (NCCLS protocol). Bacterial cultures (e.g., Staphylococcus aureus) are standardized to 1.0 × 10⁵ CFU/mL in Mueller Hinton broth. Compound dilutions (1000–7.8125 µg/mL) are tested with 10% DMSO as a negative control. MIC is determined after 24-hour incubation at 37°C by observing turbidity .
Q. What spectroscopic techniques are essential for characterizing this compound and its derivatives?
- Methodological Answer : Nuclear Magnetic Resonance (NMR) and Infrared (IR) spectroscopy are critical. NMR identifies substituent patterns (e.g., aromatic protons in Schiff base derivatives), while IR confirms functional groups like C=O (1700–1750 cm⁻¹) and C-Cl bonds (600–800 cm⁻¹). X-ray crystallography further resolves crystal packing and bond angles .
Advanced Research Questions
Q. How does structural modification (e.g., substituent variation) influence bioactivity and electronic properties?
- Methodological Answer : Systematic substitution of aldehydes (e.g., thiophene vs. halogenated benzaldehyde) alters electron density and steric effects. Density Functional Theory (DFT) calculations can model HOMO-LUMO gaps to predict reactivity. Bioactivity trends (e.g., enhanced MIC with electron-deficient substituents) are validated via comparative assays .
Q. What mechanistic insights explain discrepancies in antibacterial activity across bacterial strains?
- Methodological Answer : Contradictions in MIC data may arise from bacterial membrane permeability differences (e.g., Gram-positive vs. Gram-negative). Lipophilicity (logP) measurements and molecular docking studies can clarify interactions with bacterial targets (e.g., penicillin-binding proteins). Cross-referencing with proteomic data identifies resistance mechanisms .
Q. How can X-ray crystallography and computational modeling elucidate the compound’s role in organic electro-luminescent materials?
- Methodological Answer : Single-crystal X-ray diffraction (113 K, R-factor <0.05) reveals planar geometry and π-π stacking, critical for charge transport in electroluminescence. Time-Dependent DFT (TD-DFT) simulates excited-state properties, while UV-Vis and fluorescence spectroscopy validate emission spectra .
Q. What theoretical frameworks guide the design of derivatives for targeted applications?
- Methodological Answer : Structure-Activity Relationship (SAR) models integrate electronic (Hammett constants) and steric parameters (Taft indices). For materials science, Marcus theory predicts electron-transfer kinetics. Frameworks like Molecular Orbital Theory rationalize substituent effects on luminescence efficiency .
Q. How can researchers address synthetic challenges, such as regioselectivity in halogenated isoindole systems?
- Methodological Answer : Regioselectivity is controlled via directing groups (e.g., amino substituents) and reaction kinetics. Monitoring intermediates via LC-MS identifies competing pathways. Solvent polarity adjustments (e.g., DMF vs. THF) and catalytic additives (e.g., Lewis acids) enhance desired product formation .
Properties
CAS No. |
67887-47-2 |
---|---|
Molecular Formula |
C16Cl8N2O4 |
Molecular Weight |
567.8 g/mol |
IUPAC Name |
4,5,6,7-tetrachloro-2-(4,5,6,7-tetrachloro-1,3-dioxoisoindol-2-yl)isoindole-1,3-dione |
InChI |
InChI=1S/C16Cl8N2O4/c17-5-1-2(6(18)10(22)9(5)21)14(28)25(13(1)27)26-15(29)3-4(16(26)30)8(20)12(24)11(23)7(3)19 |
InChI Key |
JFEBIVJTGIPNKL-UHFFFAOYSA-N |
Canonical SMILES |
C12=C(C(=C(C(=C1Cl)Cl)Cl)Cl)C(=O)N(C2=O)N3C(=O)C4=C(C3=O)C(=C(C(=C4Cl)Cl)Cl)Cl |
Origin of Product |
United States |
Disclaimer and Information on In-Vitro Research Products
Please be aware that all articles and product information presented on BenchChem are intended solely for informational purposes. The products available for purchase on BenchChem are specifically designed for in-vitro studies, which are conducted outside of living organisms. In-vitro studies, derived from the Latin term "in glass," involve experiments performed in controlled laboratory settings using cells or tissues. It is important to note that these products are not categorized as medicines or drugs, and they have not received approval from the FDA for the prevention, treatment, or cure of any medical condition, ailment, or disease. We must emphasize that any form of bodily introduction of these products into humans or animals is strictly prohibited by law. It is essential to adhere to these guidelines to ensure compliance with legal and ethical standards in research and experimentation.