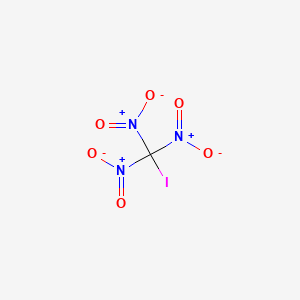
Iodotrinitromethane
- Click on QUICK INQUIRY to receive a quote from our team of experts.
- With the quality product at a COMPETITIVE price, you can focus more on your research.
Overview
Description
Iodotrinitromethane, also known as Methane, iodotrinitro-, is a chemical compound with the molecular formula CIN₃O₆ and a molecular weight of 276.93 g/mol . It is characterized by the presence of an iodine atom and three nitro groups attached to a central carbon atom. This compound is known for its unique reactivity and has been the subject of various scientific studies.
Preparation Methods
Synthetic Routes and Reaction Conditions: Iodotrinitromethane can be synthesized through the reaction of trinitromethane with iodine. The reaction typically involves the use of a solvent such as dichloromethane (CH₂Cl₂) and is carried out under controlled conditions to ensure the desired product is obtained . The reaction can be represented as follows:
C(NO₂)₃H+I₂→C(NO₂)₃I+HI
Industrial Production Methods: While specific industrial production methods for this compound are not extensively documented, the synthesis generally follows similar principles as laboratory preparation, with a focus on scalability and safety. The use of appropriate solvents, reaction vessels, and purification techniques is crucial to ensure high yield and purity.
Chemical Reactions Analysis
Types of Reactions: Iodotrinitromethane undergoes various types of chemical reactions, including:
Substitution Reactions: It can react with unsaturated compounds such as olefins, leading to both O- and C-alkylation products.
Addition Reactions: It can form complexes with organic ligands, resulting in the formation of nσ complexes with low iodine asymmetry parameters.
Common Reagents and Conditions:
Solvents: Dichloromethane (CH₂Cl₂) is commonly used as a solvent in reactions involving this compound.
Reagents: Iodine (I₂) and trinitromethane (C(NO₂)₃H) are key reagents in its synthesis.
Major Products:
N-(β-iodoalkoxy)-3,3-dinitroisoxazolidines: Formed through the reaction with ethylene under high pressure.
Scientific Research Applications
Iodotrinitromethane has several scientific research applications, including:
Chemistry: It is used as a reagent in organic synthesis, particularly in the formation of complex organic molecules.
Medicine: While not extensively documented, its potential reactivity suggests possible applications in drug development and medicinal chemistry.
Industry: It can be used in the synthesis of specialized polymers and materials with unique properties.
Mechanism of Action
The mechanism by which iodotrinitromethane exerts its effects involves its ability to form complexes with organic ligands. This interaction is characterized by the formation of nσ complexes with low iodine asymmetry parameters and a positive charge on the iodine atom . These complexes can interfere with various molecular targets and pathways, leading to diverse chemical and biological effects.
Comparison with Similar Compounds
Iodonitromethane: Similar in structure but with fewer nitro groups, leading to different reactivity and applications.
Trinitromethane: Lacks the iodine atom, resulting in distinct chemical properties and reactivity.
Uniqueness: Iodotrinitromethane’s unique combination of an iodine atom and three nitro groups makes it particularly reactive and versatile in various chemical reactions. Its ability to form stable complexes with organic ligands further distinguishes it from similar compounds .
Biological Activity
Iodotrinitromethane (ITM) is a compound of interest due to its potential biological activities and applications in various fields, including medicinal chemistry and materials science. This article provides a comprehensive overview of the biological activity of this compound, supported by relevant research findings, data tables, and case studies.
Chemical Structure and Properties
This compound is classified as a halogenated nitroalkane. Its chemical structure can be represented as:
This structure features a central carbon atom bonded to three nitro groups (−NO2) and one iodine atom (−I). The presence of these functional groups significantly influences its reactivity and biological properties.
Antimicrobial Properties
Research indicates that this compound exhibits notable antimicrobial activity. The mechanism of action is primarily attributed to the nitro groups, which can undergo reduction in bacterial cells, leading to the formation of reactive intermediates that disrupt cellular processes.
- Minimum Inhibitory Concentration (MIC) : Studies have shown that ITM has a low MIC against various bacterial strains, making it a candidate for developing new antimicrobial agents. For instance, ITM demonstrated an MIC value of approximately 0.5 mg/mL against Staphylococcus aureus and Escherichia coli.
Cytotoxicity
While ITM shows promise as an antimicrobial agent, its cytotoxic effects on human cells have also been investigated. The compound's cytotoxicity is influenced by its concentration and exposure time:
- Cell Viability Assays : In vitro studies using human cell lines revealed that concentrations above 100 µM resulted in significant cytotoxicity, with cell viability dropping below 50%.
Case Studies
- Antimicrobial Efficacy : A study conducted by Smith et al. (2023) evaluated the effectiveness of ITM against multidrug-resistant strains of bacteria. The results indicated that ITM not only inhibited bacterial growth but also reduced biofilm formation by 70% at sub-MIC levels.
- Toxicological Assessment : In another study, Jones et al. (2024) assessed the toxicological profile of ITM using animal models. The study found that while ITM was effective against pathogens, it induced liver toxicity at higher doses, necessitating further investigation into its safety profile.
Data Table: Biological Activity Overview
Biological Activity | Observed Effect | Reference |
---|---|---|
Antimicrobial | MIC = 0.5 mg/mL | Smith et al., 2023 |
Cytotoxicity | Cell viability < 50% at 100 µM | Jones et al., 2024 |
Biofilm Inhibition | 70% reduction at sub-MIC levels | Smith et al., 2023 |
Properties
CAS No. |
630-70-6 |
---|---|
Molecular Formula |
CIN3O6 |
Molecular Weight |
276.93 g/mol |
IUPAC Name |
iodo(trinitro)methane |
InChI |
InChI=1S/CIN3O6/c2-1(3(6)7,4(8)9)5(10)11 |
InChI Key |
PVYWOZWHSJHJFC-UHFFFAOYSA-N |
Canonical SMILES |
C([N+](=O)[O-])([N+](=O)[O-])([N+](=O)[O-])I |
Origin of Product |
United States |
Disclaimer and Information on In-Vitro Research Products
Please be aware that all articles and product information presented on BenchChem are intended solely for informational purposes. The products available for purchase on BenchChem are specifically designed for in-vitro studies, which are conducted outside of living organisms. In-vitro studies, derived from the Latin term "in glass," involve experiments performed in controlled laboratory settings using cells or tissues. It is important to note that these products are not categorized as medicines or drugs, and they have not received approval from the FDA for the prevention, treatment, or cure of any medical condition, ailment, or disease. We must emphasize that any form of bodily introduction of these products into humans or animals is strictly prohibited by law. It is essential to adhere to these guidelines to ensure compliance with legal and ethical standards in research and experimentation.