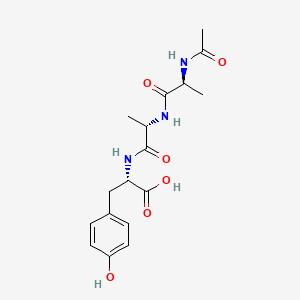
Acetyl-alanyl-alanyl-tyrosine
- Click on QUICK INQUIRY to receive a quote from our team of experts.
- With the quality product at a COMPETITIVE price, you can focus more on your research.
Overview
Description
Acetyl-alanyl-alanyl-tyrosine is a peptide compound formed by the condensation of acetylated alanine and tyrosine. This compound is of interest due to its potential biological activities and applications in various fields such as chemistry, biology, medicine, and industry.
Preparation Methods
Synthetic Routes and Reaction Conditions
Acetyl-alanyl-alanyl-tyrosine can be synthesized through a series of peptide coupling reactions. The synthesis typically involves the use of protected amino acids to prevent unwanted side reactions. The general steps include:
Protection of Amino Groups: The amino groups of alanine and tyrosine are protected using suitable protecting groups such as Boc (tert-butyloxycarbonyl) or Fmoc (9-fluorenylmethoxycarbonyl).
Coupling Reactions: The protected amino acids are coupled using peptide coupling reagents like EDC (1-ethyl-3-(3-dimethylaminopropyl)carbodiimide) or DCC (dicyclohexylcarbodiimide) in the presence of a base such as DIPEA (N,N-diisopropylethylamine).
Deprotection: The protecting groups are removed under acidic or basic conditions to yield the desired peptide.
Industrial Production Methods
Industrial production of this compound may involve solid-phase peptide synthesis (SPPS) techniques, which allow for the efficient and scalable production of peptides. SPPS involves the stepwise addition of protected amino acids to a solid resin, followed by cleavage and purification of the final product.
Chemical Reactions Analysis
Types of Reactions
Acetyl-alanyl-alanyl-tyrosine can undergo various chemical reactions, including:
Hydrolysis: The peptide bonds can be hydrolyzed under acidic or basic conditions to yield the constituent amino acids.
Oxidation: The tyrosine residue can undergo oxidation to form dopaquinone, which can further react to form melanin-like compounds.
Substitution: The acetyl group can be substituted with other acyl groups through nucleophilic acyl substitution reactions.
Common Reagents and Conditions
Hydrolysis: Acidic (HCl) or basic (NaOH) conditions.
Oxidation: Oxidizing agents such as hydrogen peroxide (H2O2) or potassium permanganate (KMnO4).
Substitution: Nucleophiles such as amines or thiols in the presence of a base.
Major Products Formed
Hydrolysis: Alanine, tyrosine, and acetic acid.
Oxidation: Dopaquinone and melanin-like compounds.
Substitution: Various acylated derivatives depending on the nucleophile used.
Scientific Research Applications
Acetyl-alanyl-alanyl-tyrosine has several scientific research applications:
Chemistry: Used as a model compound for studying peptide synthesis and reactions.
Biology: Investigated for its role in protein synthesis and enzymatic reactions.
Medicine: Explored for its potential therapeutic effects, including its ability to promote melanin synthesis and its use in drug delivery systems.
Industry: Utilized in the production of peptide-based materials and as a component in cosmetic formulations.
Mechanism of Action
The mechanism of action of Acetyl-alanyl-alanyl-tyrosine involves its interaction with specific molecular targets and pathways. For example, the tyrosine residue can be converted to dopaquinone, which plays a role in melanin synthesis. Additionally, the peptide may interact with enzymes and receptors involved in protein synthesis and cellular signaling pathways.
Comparison with Similar Compounds
Similar Compounds
N-Acetyltyrosine: Used as a high solubility precursor to tyrosine.
N-Acylphenylalanine: Another N-acylated aromatic amino acid with similar properties.
N-Acyltryptophan: Shares structural similarities and potential biological activities.
Uniqueness
Acetyl-alanyl-alanyl-tyrosine is unique due to its specific sequence and the presence of both acetylated alanine and tyrosine residues. This combination imparts distinct physicochemical properties and biological activities, making it a valuable compound for various applications.
Biological Activity
Acetyl-alanyl-alanyl-tyrosine (Ac-Ala-Ala-Tyr) is a synthetic dipeptide that has garnered attention due to its potential biological activities, particularly in the context of neuroprotection, stress response, and metabolic regulation. This article presents a comprehensive overview of its biological activity, supported by relevant case studies and research findings.
Chemical Structure and Synthesis
This compound is derived from the amino acids alanine and tyrosine, with an acetyl group enhancing its stability and bioavailability. The synthesis typically involves standard peptide coupling techniques, often utilizing activated forms of the amino acids to facilitate the formation of peptide bonds.
Stress Response Modulation
N-acetyl-L-tyrosine has been shown to influence neurotransmitter synthesis, particularly dopamine and norepinephrine, which are critical for stress response . Ac-Ala-Ala-Tyr may also play a role in this pathway, promoting cognitive function under stress conditions. Studies have indicated that supplementation with tyrosine derivatives can mitigate cognitive decline during stressful situations .
Metabolic Implications
The metabolism of Ac-Ala-Ala-Tyr is expected to release free tyrosine upon hydrolysis. This release can be significant in conditions such as hepatic failure, where traditional sources of tyrosine may not be effectively utilized. Research on related dipeptides like L-alanyl-L-tyrosine (Ala-Tyr) shows that these compounds can be rapidly hydrolyzed and utilized for protein synthesis and energy production in both healthy individuals and those with liver impairments .
Case Studies
- Cognitive Performance Under Stress : A clinical trial investigated the effects of N-acetyl-L-tyrosine on cognitive performance during acute stress. Participants who received NALT showed improved memory recall compared to placebo groups, suggesting that acetylated forms of tyrosine can enhance cognitive resilience under pressure .
- Hepatic Function : In patients with hepatic failure, the elimination rates of Ala-Tyr were comparable to healthy controls, indicating that dipeptides can serve as effective sources of tyrosine even when liver function is compromised . This suggests that Ac-Ala-Ala-Tyr could similarly support metabolic needs in such populations.
Comparative Analysis of Dipeptides
The following table summarizes key findings related to the biological activity of various tyrosine-containing dipeptides:
Dipeptide | Neuroprotective Effects | Stress Response | Metabolic Utilization |
---|---|---|---|
Acetyl-alanylalany-tyrosine (Ac-Ala-Ala-Tyr) | Potentially beneficial (similar structure to NAT) | May enhance cognitive function under stress | Expected rapid hydrolysis to tyrosine |
N-acetyl-L-tyrosine (NAT) | Confirmed neuroprotective effects | Improved cognitive performance under stress | Effective source of tyrosine in parenteral nutrition |
L-alanyl-L-tyrosine (Ala-Tyr) | Limited studies; potential based on structure | Supports neurotransmitter synthesis | Rapidly hydrolyzed; effective in hepatic dysfunction |
Properties
CAS No. |
75795-03-8 |
---|---|
Molecular Formula |
C17H23N3O6 |
Molecular Weight |
365.4 g/mol |
IUPAC Name |
(2S)-2-[[(2S)-2-[[(2S)-2-acetamidopropanoyl]amino]propanoyl]amino]-3-(4-hydroxyphenyl)propanoic acid |
InChI |
InChI=1S/C17H23N3O6/c1-9(18-11(3)21)15(23)19-10(2)16(24)20-14(17(25)26)8-12-4-6-13(22)7-5-12/h4-7,9-10,14,22H,8H2,1-3H3,(H,18,21)(H,19,23)(H,20,24)(H,25,26)/t9-,10-,14-/m0/s1 |
InChI Key |
JJPMVRHZSQGALO-BHDSKKPTSA-N |
Isomeric SMILES |
C[C@@H](C(=O)N[C@@H](C)C(=O)N[C@@H](CC1=CC=C(C=C1)O)C(=O)O)NC(=O)C |
Canonical SMILES |
CC(C(=O)NC(C)C(=O)NC(CC1=CC=C(C=C1)O)C(=O)O)NC(=O)C |
Origin of Product |
United States |
Disclaimer and Information on In-Vitro Research Products
Please be aware that all articles and product information presented on BenchChem are intended solely for informational purposes. The products available for purchase on BenchChem are specifically designed for in-vitro studies, which are conducted outside of living organisms. In-vitro studies, derived from the Latin term "in glass," involve experiments performed in controlled laboratory settings using cells or tissues. It is important to note that these products are not categorized as medicines or drugs, and they have not received approval from the FDA for the prevention, treatment, or cure of any medical condition, ailment, or disease. We must emphasize that any form of bodily introduction of these products into humans or animals is strictly prohibited by law. It is essential to adhere to these guidelines to ensure compliance with legal and ethical standards in research and experimentation.