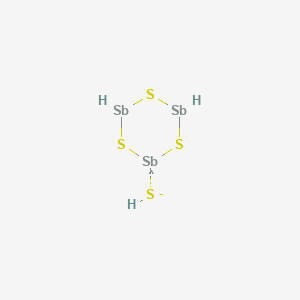
Triantimony tetrasulfide
- Click on QUICK INQUIRY to receive a quote from our team of experts.
- With the quality product at a COMPETITIVE price, you can focus more on your research.
Overview
Description
Triantimony tetrasulfide is an inorganic compound with the chemical formula ( \text{Sb}_3\text{S}_4 ). It is known for its unique properties and applications in various fields, including chemistry, biology, and industry. This compound is characterized by its distinct molecular structure and reactivity, making it a subject of interest in scientific research.
Preparation Methods
Synthetic Routes and Reaction Conditions
Triantimony tetrasulfide can be synthesized through various methods. One common synthetic route involves the direct combination of antimony and sulfur at elevated temperatures. The reaction can be represented as: [ 3 \text{Sb} + 4 \text{S} \rightarrow \text{Sb}_3\text{S}_4 ] This reaction typically requires a controlled environment to ensure the proper stoichiometry and to prevent the formation of unwanted by-products.
Industrial Production Methods
In industrial settings, this compound is produced using high-temperature furnaces where antimony and sulfur are combined under controlled conditions. The process involves careful monitoring of temperature and pressure to optimize yield and purity. The resulting product is then purified through various techniques, such as sublimation or recrystallization, to obtain high-purity this compound.
Chemical Reactions Analysis
Types of Reactions
Triantimony tetrasulfide undergoes several types of chemical reactions, including:
Oxidation: When exposed to oxygen, this compound can oxidize to form antimony oxides and sulfur dioxide.
Reduction: It can be reduced by strong reducing agents to yield elemental antimony and hydrogen sulfide.
Substitution: In the presence of halogens, substitution reactions can occur, leading to the formation of antimony halides and sulfur.
Common Reagents and Conditions
Oxidation: Oxygen or air at elevated temperatures.
Reduction: Hydrogen gas or other reducing agents at high temperatures.
Substitution: Halogens such as chlorine or bromine under controlled conditions.
Major Products Formed
Oxidation: Antimony oxides (( \text{Sb}_2\text{O}_3 ), ( \text{Sb}_2\text{O}_5 )) and sulfur dioxide (( \text{SO}_2 )).
Reduction: Elemental antimony (( \text{Sb} )) and hydrogen sulfide (( \text{H}_2\text{S} )).
Substitution: Antimony halides (( \text{SbCl}_3 ), ( \text{SbBr}_3 )) and elemental sulfur (( \text{S} )).
Scientific Research Applications
Triantimony tetrasulfide has a wide range of applications in scientific research:
Chemistry: It is used as a precursor for the synthesis of other antimony compounds and as a reagent in various chemical reactions.
Biology: Research has explored its potential use in biological systems, particularly in the study of its interactions with biomolecules.
Medicine: Investigations are ongoing into its potential therapeutic applications, including its use in drug delivery systems and as an antimicrobial agent.
Industry: It is used in the production of semiconductors, pigments, and as a flame retardant in various materials.
Mechanism of Action
The mechanism by which triantimony tetrasulfide exerts its effects involves its interaction with molecular targets and pathways. In biological systems, it can interact with proteins and enzymes, potentially inhibiting their activity. Its antimicrobial properties are attributed to its ability to disrupt cellular processes in microorganisms. In industrial applications, its flame-retardant properties are due to its ability to form a protective barrier that prevents the spread of flames.
Comparison with Similar Compounds
Similar Compounds
Tetrasulfur tetranitride (( \text{S}_4\text{N}_4 )): Known for its explosive properties and unique structure.
Tetraarsenic tetrasulfide (( \text{As}_4\text{S}_4 )): Used in traditional medicine and as a pigment.
Vanadium tetrasulfide (( \text{VS}_4 )): Exhibits peroxidase-like activity and is used in catalytic applications.
Uniqueness of Triantimony Tetrasulfide
This compound is unique due to its specific combination of antimony and sulfur, which imparts distinct chemical and physical properties. Its applications in various fields, from industrial to biological, highlight its versatility and importance in scientific research.
Properties
CAS No. |
61349-45-9 |
---|---|
Molecular Formula |
H3S4Sb3- |
Molecular Weight |
496.6 g/mol |
InChI |
InChI=1S/H2S.3S.3Sb.2H/h1H2;;;;;;;;/p-1 |
InChI Key |
FGCXRVJLPGCLJJ-UHFFFAOYSA-M |
Canonical SMILES |
[SH-].S1[SbH]S[Sb]S[SbH]1 |
Origin of Product |
United States |
Disclaimer and Information on In-Vitro Research Products
Please be aware that all articles and product information presented on BenchChem are intended solely for informational purposes. The products available for purchase on BenchChem are specifically designed for in-vitro studies, which are conducted outside of living organisms. In-vitro studies, derived from the Latin term "in glass," involve experiments performed in controlled laboratory settings using cells or tissues. It is important to note that these products are not categorized as medicines or drugs, and they have not received approval from the FDA for the prevention, treatment, or cure of any medical condition, ailment, or disease. We must emphasize that any form of bodily introduction of these products into humans or animals is strictly prohibited by law. It is essential to adhere to these guidelines to ensure compliance with legal and ethical standards in research and experimentation.