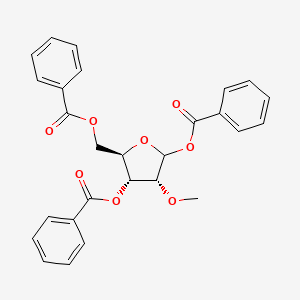
1,3,5-Tri-O-benzoyl-2-O-methyl-D-ribofuranose
Overview
Description
1,3,5-Tri-O-benzoyl-2-O-methyl-D-ribofuranose is a chemical compound derived from ribose, a type of sugar. This compound is characterized by the presence of three benzoyl groups and one methyl group attached to the ribofuranose ring. It is commonly used as an intermediate in the synthesis of nucleosides and other biologically active molecules .
Mechanism of Action
Target of Action
It is known that this compound is a purine nucleoside analog . Purine nucleoside analogs are known to target various enzymes involved in DNA synthesis and repair, thereby interfering with the replication and survival of cells, particularly cancer cells .
Mode of Action
As a purine nucleoside analog, it likely incorporates into dna during replication, causing premature termination of the growing dna strand . This results in the inhibition of DNA synthesis and induction of apoptosis .
Biochemical Pathways
Purine nucleoside analogs generally interfere with the dna synthesis pathway, leading to cell cycle arrest and apoptosis .
Result of Action
As a purine nucleoside analog, it is expected to cause dna damage, leading to cell cycle arrest and apoptosis, particularly in rapidly dividing cells such as cancer cells .
Preparation Methods
Synthetic Routes and Reaction Conditions
The synthesis of 1,3,5-Tri-O-benzoyl-2-O-methyl-D-ribofuranose typically involves the protection of hydroxyl groups on the ribose molecule followed by methylation. One common method involves the use of benzoyl chloride and a base to introduce the benzoyl groups. The methylation is often achieved using methyl iodide in the presence of a base .
Industrial Production Methods
Industrial production of this compound follows similar synthetic routes but on a larger scale. The process involves careful control of reaction conditions such as temperature, pH, and reaction time to ensure high yield and purity. The use of automated reactors and continuous flow systems can enhance the efficiency and scalability of the production process .
Chemical Reactions Analysis
Types of Reactions
1,3,5-Tri-O-benzoyl-2-O-methyl-D-ribofuranose undergoes various chemical reactions, including:
Oxidation: This compound can be oxidized to form corresponding carboxylic acids.
Reduction: Reduction reactions can convert the benzoyl groups to hydroxyl groups.
Substitution: The benzoyl groups can be substituted with other functional groups under appropriate conditions
Common Reagents and Conditions
Oxidation: Common oxidizing agents include potassium permanganate and chromium trioxide.
Reduction: Reducing agents such as lithium aluminum hydride or sodium borohydride are used.
Substitution: Reagents like sodium methoxide or sodium ethoxide can facilitate substitution reactions
Major Products Formed
The major products formed from these reactions depend on the specific conditions and reagents used. For example, oxidation can yield carboxylic acids, while reduction can produce hydroxylated derivatives .
Scientific Research Applications
1,3,5-Tri-O-benzoyl-2-O-methyl-D-ribofuranose has several applications in scientific research:
Chemistry: It is used as an intermediate in the synthesis of nucleosides and other complex molecules.
Biology: The compound is employed in the study of nucleic acid analogs and their interactions with biological systems.
Medicine: It serves as a precursor in the development of antiviral and anticancer drugs.
Industry: The compound is used in the production of pharmaceuticals and fine chemicals
Comparison with Similar Compounds
Similar Compounds
1,3,5-Tri-O-benzoyl-D-ribofuranose: Similar structure but lacks the methyl group.
1-O-Acetyl-2,3,5-tri-O-benzoyl-beta-D-ribofuranose: Another ribose-derived compound used in nucleoside synthesis .
Uniqueness
1,3,5-Tri-O-benzoyl-2-O-methyl-D-ribofuranose is unique due to the presence of the methyl group, which can influence its reactivity and biological activity. This structural difference can lead to variations in its interactions with enzymes and other biological molecules, making it a valuable compound for specific applications .
Properties
IUPAC Name |
[(2R,3R,4R)-3,5-dibenzoyloxy-4-methoxyoxolan-2-yl]methyl benzoate | |
---|---|---|
Source | PubChem | |
URL | https://pubchem.ncbi.nlm.nih.gov | |
Description | Data deposited in or computed by PubChem | |
InChI |
InChI=1S/C27H24O8/c1-31-23-22(34-25(29)19-13-7-3-8-14-19)21(17-32-24(28)18-11-5-2-6-12-18)33-27(23)35-26(30)20-15-9-4-10-16-20/h2-16,21-23,27H,17H2,1H3/t21-,22-,23-,27?/m1/s1 | |
Source | PubChem | |
URL | https://pubchem.ncbi.nlm.nih.gov | |
Description | Data deposited in or computed by PubChem | |
InChI Key |
BTKQRBSDABCRCX-IANNTBFTSA-N | |
Source | PubChem | |
URL | https://pubchem.ncbi.nlm.nih.gov | |
Description | Data deposited in or computed by PubChem | |
Canonical SMILES |
COC1C(C(OC1OC(=O)C2=CC=CC=C2)COC(=O)C3=CC=CC=C3)OC(=O)C4=CC=CC=C4 | |
Source | PubChem | |
URL | https://pubchem.ncbi.nlm.nih.gov | |
Description | Data deposited in or computed by PubChem | |
Isomeric SMILES |
CO[C@@H]1[C@@H]([C@H](OC1OC(=O)C2=CC=CC=C2)COC(=O)C3=CC=CC=C3)OC(=O)C4=CC=CC=C4 | |
Source | PubChem | |
URL | https://pubchem.ncbi.nlm.nih.gov | |
Description | Data deposited in or computed by PubChem | |
Molecular Formula |
C27H24O8 | |
Source | PubChem | |
URL | https://pubchem.ncbi.nlm.nih.gov | |
Description | Data deposited in or computed by PubChem | |
Molecular Weight |
476.5 g/mol | |
Source | PubChem | |
URL | https://pubchem.ncbi.nlm.nih.gov | |
Description | Data deposited in or computed by PubChem | |
Retrosynthesis Analysis
AI-Powered Synthesis Planning: Our tool employs the Template_relevance Pistachio, Template_relevance Bkms_metabolic, Template_relevance Pistachio_ringbreaker, Template_relevance Reaxys, Template_relevance Reaxys_biocatalysis model, leveraging a vast database of chemical reactions to predict feasible synthetic routes.
One-Step Synthesis Focus: Specifically designed for one-step synthesis, it provides concise and direct routes for your target compounds, streamlining the synthesis process.
Accurate Predictions: Utilizing the extensive PISTACHIO, BKMS_METABOLIC, PISTACHIO_RINGBREAKER, REAXYS, REAXYS_BIOCATALYSIS database, our tool offers high-accuracy predictions, reflecting the latest in chemical research and data.
Strategy Settings
Precursor scoring | Relevance Heuristic |
---|---|
Min. plausibility | 0.01 |
Model | Template_relevance |
Template Set | Pistachio/Bkms_metabolic/Pistachio_ringbreaker/Reaxys/Reaxys_biocatalysis |
Top-N result to add to graph | 6 |
Feasible Synthetic Routes
Disclaimer and Information on In-Vitro Research Products
Please be aware that all articles and product information presented on BenchChem are intended solely for informational purposes. The products available for purchase on BenchChem are specifically designed for in-vitro studies, which are conducted outside of living organisms. In-vitro studies, derived from the Latin term "in glass," involve experiments performed in controlled laboratory settings using cells or tissues. It is important to note that these products are not categorized as medicines or drugs, and they have not received approval from the FDA for the prevention, treatment, or cure of any medical condition, ailment, or disease. We must emphasize that any form of bodily introduction of these products into humans or animals is strictly prohibited by law. It is essential to adhere to these guidelines to ensure compliance with legal and ethical standards in research and experimentation.