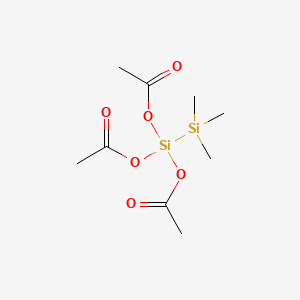
2,2,2-Trimethyldisilane-1,1,1-triyl triacetate
- Click on QUICK INQUIRY to receive a quote from our team of experts.
- With the quality product at a COMPETITIVE price, you can focus more on your research.
Overview
Description
2,2,2-Trimethyldisilane-1,1,1-triyl triacetate: is a chemical compound with the molecular formula C9H18O6Si2 and a molecular weight of 278.41 g/mol . This compound is known for its unique structure, which includes two silicon atoms bonded to three acetate groups. It is used in various scientific and industrial applications due to its distinctive chemical properties.
Preparation Methods
Synthetic Routes and Reaction Conditions: The synthesis of 2,2,2-Trimethyldisilane-1,1,1-triyl triacetate typically involves the reaction of trimethylchlorosilane with acetic anhydride in the presence of a catalyst. The reaction is carried out under controlled temperature and pressure conditions to ensure the formation of the desired product .
Industrial Production Methods: In industrial settings, the production of this compound follows a similar synthetic route but on a larger scale. The process involves the use of large reactors and precise control of reaction parameters to achieve high yields and purity of the compound .
Chemical Reactions Analysis
Types of Reactions: 2,2,2-Trimethyldisilane-1,1,1-triyl triacetate undergoes various chemical reactions, including:
Oxidation: The compound can be oxidized to form silanols and siloxanes.
Reduction: Reduction reactions can convert the acetate groups to alcohols.
Substitution: The acetate groups can be substituted with other functional groups under appropriate conditions.
Common Reagents and Conditions:
Oxidation: Common oxidizing agents include hydrogen peroxide and potassium permanganate.
Reduction: Reducing agents such as lithium aluminum hydride are used.
Substitution: Substitution reactions often require catalysts like palladium or platinum.
Major Products:
Oxidation: Silanols and siloxanes.
Reduction: Trimethylsilanetriol.
Substitution: Various substituted silanes depending on the reagents used.
Scientific Research Applications
Chemical Properties and Structure
The compound is characterized by its silane backbone and triacetate functional groups, which contribute to its reactivity and versatility in chemical reactions. The presence of silicon atoms allows for the formation of siloxane bonds, which are crucial in many applications.
Surface Modification
One of the primary applications of 2,2,2-Trimethyldisilane-1,1,1-triyl triacetate is in the modification of surfaces to enhance their properties. This compound can be used to create hydrophobic surfaces by forming a silane layer that repels water. Such modifications are beneficial in various industries:
- Coatings: Improved resistance to moisture and corrosion.
- Textiles: Enhanced water repellency.
- Electronics: Protection of components from environmental damage.
Adhesives and Sealants
Due to its ability to form strong bonds with various substrates, this compound is utilized in the formulation of adhesives and sealants. The silane groups facilitate adhesion to metals, glass, and plastics, making it valuable in construction and automotive applications.
Silylation Reactions
In organic chemistry, this compound serves as a silylating agent. It is employed in the protection of hydroxyl groups during synthesis processes:
- Silyl Ether Formation: Protects alcohols from undesired reactions during multi-step syntheses.
- Deprotection Strategies: The triacetate groups can be selectively removed under mild conditions to regenerate the original alcohol.
Nanocomposite Materials
The compound is also explored for use in nanocomposites where it can enhance the mechanical properties and thermal stability of polymer matrices. Its incorporation into polymer blends leads to:
- Improved Strength: By reinforcing the polymer structure at the nanoscale.
- Thermal Resistance: Enhancing performance under elevated temperatures.
Synthesis of Nanoparticles
In nanoparticle synthesis, this compound can act as a precursor for silicon-based nanoparticles. These nanoparticles have potential applications in:
- Drug Delivery Systems: Due to their biocompatibility.
- Photovoltaic Cells: Enhancing light absorption and conversion efficiency.
Case Study: Surface Coating Applications
A study demonstrated that applying a silane coating using this compound significantly improved the water repellency of glass substrates compared to untreated surfaces. The treated surfaces exhibited a contact angle greater than 100 degrees, indicating excellent hydrophobic properties.
Case Study: Organic Synthesis Efficiency
Research indicated that using this compound as a silylating agent resulted in higher yields during the synthesis of complex organic molecules compared to traditional methods. The ease of deprotection allowed for streamlined synthetic pathways.
Data Table: Summary of Applications
Application Area | Specific Use | Benefits |
---|---|---|
Materials Science | Surface modification | Enhanced hydrophobicity |
Adhesives and sealants | Stronger bonding with substrates | |
Organic Synthesis | Silylation reactions | Protection of functional groups |
Nanotechnology | Nanocomposite materials | Improved mechanical properties |
Synthesis of nanoparticles | Applications in drug delivery and photovoltaics |
Mechanism of Action
The mechanism of action of 2,2,2-Trimethyldisilane-1,1,1-triyl triacetate involves its interaction with various molecular targets and pathways. The compound’s silicon atoms can form stable bonds with other elements, allowing it to act as a cross-linking agent in polymers and other materials. Additionally, the acetate groups can undergo hydrolysis to release acetic acid, which can further participate in chemical reactions .
Comparison with Similar Compounds
- 2,2,2-Trimethyl-1,1,1-disilanetriol triacetate
- 1,1,1-Disilanetriol, 2,2,2-trimethyl-, triacetate (9CI)
Comparison: 2,2,2-Trimethyldisilane-1,1,1-triyl triacetate is unique due to its specific arrangement of silicon and acetate groups, which imparts distinct chemical properties. Compared to similar compounds, it offers enhanced stability and reactivity, making it suitable for a broader range of applications in scientific research and industry .
Q & A
Q. What are the established synthetic routes for 2,2,2-Trimethyldisilane-1,1,1-triyl triacetate, and what reaction conditions optimize yield?
Basic
The synthesis typically involves acetylation of a disilane precursor. A common method is reacting 2,2,2-trimethyldisilanol with acetic anhydride in the presence of an acid catalyst (e.g., sulfuric acid) under anhydrous conditions. Optimization includes controlling stoichiometry (3:1 molar ratio of acetic anhydride to silanol), inert atmosphere (N₂/Ar), and purification via fractional distillation or silica gel chromatography . Yield improvements may involve stepwise acetylation to minimize side reactions like oligomerization.
Q. Which spectroscopic techniques are most effective for characterizing the structure of this compound?
Basic
Key techniques include:
- ²⁹Si NMR : To confirm the disilane backbone and methyl/acetyl group attachment (e.g., δ −10 to −20 ppm for Si–OAc).
- ¹H/¹³C NMR : For methyl (δ 0.1–0.3 ppm) and acetate (δ 2.0–2.1 ppm for CH₃COO) groups.
- IR Spectroscopy : Strong C=O stretches (~1740 cm⁻¹) and Si–O vibrations (~1050 cm⁻¹).
- Mass Spectrometry (HRMS) : To verify molecular weight (e.g., [M+H]⁺ or [M+Na]⁺ adducts).
Cross-validation with elemental analysis ensures purity .
Q. How does the steric environment of the trimethyl disilane core influence the reactivity of the triacetate groups in nucleophilic substitution reactions?
Advanced
The bulky trimethyl groups hinder nucleophilic attack at silicon, directing reactivity toward the acetate moieties. Methodological approaches include:
- Kinetic Studies : Comparing reaction rates with less hindered analogs (e.g., triethyl tricarboxylate) under identical conditions.
- Computational Modeling (DFT) : To map steric maps and transition states.
- Regioselectivity Analysis : Using labeled nucleophiles (e.g., D₂O) to track substitution sites .
Q. What are the challenges in achieving regioselective hydrolysis of the triacetate groups, and how can they be methodologically addressed?
Advanced
Challenges include simultaneous hydrolysis of all three acetates due to similar electronic environments. Strategies involve:
- pH-Controlled Hydrolysis : Using mild alkaline conditions (e.g., NaHCO₃) for partial deprotection.
- Enzymatic Catalysis : Lipases (e.g., Candida antarctica) for selective ester cleavage.
- Protecting Group Strategies : Temporarily masking one acetate to direct reactivity .
Q. What are the common impurities encountered during synthesis, and how are they identified and removed?
Basic
Impurities include:
- Partially acetylated intermediates : Detected via GC-MS or HPLC (retention time shifts).
- Oligomeric byproducts : Identified by SEC (size-exclusion chromatography) or MALDI-TOF.
Purification involves silica gel chromatography (hexane/EtOAc gradient) or recrystallization from ethanol .
Q. How can computational chemistry predict the stability and degradation pathways of this compound under thermal stress?
Advanced
- Thermogravimetric Analysis (TGA) : Paired with DFT calculations to model decomposition (e.g., acetate loss vs. Si–Si bond cleavage).
- Molecular Dynamics Simulations : To assess thermal stability thresholds (e.g., bond dissociation energies).
- Degradation Product Analysis : Using GC-MS to validate predicted pathways .
Q. What safety considerations are critical when handling this compound?
Basic
- Ventilation : Avoid inhalation of acetic acid vapors from decomposition.
- Fire Risk : Silanes may be pyrophoric; store under inert gas.
- PPE : Gloves and goggles to prevent skin/eye irritation from acetates.
Refer to SDS guidelines for silane derivatives and acetic acid analogs .
Q. In cross-coupling reactions, how does the disilane moiety compare to carbon frameworks, and what methods validate its role?
Advanced
The disilane core can act as a directing group or stabilizer in metal-catalyzed reactions (e.g., Pd-catalyzed couplings). Methodologies include:
- X-ray Diffraction (XRD) : To confirm coordination geometry with metals.
- Kinetic Isotope Effects (KIE) : Comparing Si–Si vs. C–C bond cleavage rates.
- In Situ NMR : Monitoring reaction intermediates (e.g., Si–M bonds) .
Properties
CAS No. |
53580-48-6 |
---|---|
Molecular Formula |
C9H18O6Si2 |
Molecular Weight |
278.41 g/mol |
IUPAC Name |
[diacetyloxy(trimethylsilyl)silyl] acetate |
InChI |
InChI=1S/C9H18O6Si2/c1-7(10)13-17(14-8(2)11,15-9(3)12)16(4,5)6/h1-6H3 |
InChI Key |
UDTVKJFJPHLKQE-UHFFFAOYSA-N |
Canonical SMILES |
CC(=O)O[Si](OC(=O)C)(OC(=O)C)[Si](C)(C)C |
Origin of Product |
United States |
Disclaimer and Information on In-Vitro Research Products
Please be aware that all articles and product information presented on BenchChem are intended solely for informational purposes. The products available for purchase on BenchChem are specifically designed for in-vitro studies, which are conducted outside of living organisms. In-vitro studies, derived from the Latin term "in glass," involve experiments performed in controlled laboratory settings using cells or tissues. It is important to note that these products are not categorized as medicines or drugs, and they have not received approval from the FDA for the prevention, treatment, or cure of any medical condition, ailment, or disease. We must emphasize that any form of bodily introduction of these products into humans or animals is strictly prohibited by law. It is essential to adhere to these guidelines to ensure compliance with legal and ethical standards in research and experimentation.