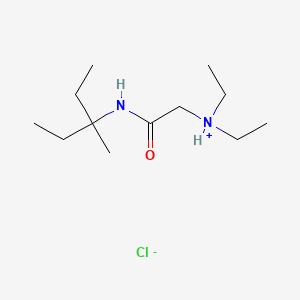
2-(Diethylamino)-N-(1-ethyl-1-methylpropyl)acetamide hydrochloride
- Click on QUICK INQUIRY to receive a quote from our team of experts.
- With the quality product at a COMPETITIVE price, you can focus more on your research.
Overview
Description
2-(Diethylamino)-N-(1-ethyl-1-methylpropyl)acetamide hydrochloride is a synthetic organic compound. It is commonly used in various chemical and pharmaceutical applications due to its unique structural properties and reactivity.
Preparation Methods
Synthetic Routes and Reaction Conditions: The synthesis of 2-(Diethylamino)-N-(1-ethyl-1-methylpropyl)acetamide hydrochloride typically involves the reaction of diethylamine with 1-ethyl-1-methylpropylamine in the presence of acetic anhydride. The reaction is carried out under controlled temperature and pressure conditions to ensure the formation of the desired product.
Industrial Production Methods: In industrial settings, the production of this compound is scaled up using continuous flow reactors. This allows for better control over reaction conditions and higher yields. The process involves the same basic reaction but is optimized for large-scale production.
Types of Reactions:
Oxidation: The compound can undergo oxidation reactions, typically in the presence of strong oxidizing agents such as potassium permanganate or chromium trioxide.
Reduction: Reduction reactions can be carried out using reducing agents like lithium aluminum hydride or sodium borohydride.
Substitution: The compound can participate in nucleophilic substitution reactions, where the diethylamino group can be replaced by other nucleophiles.
Common Reagents and Conditions:
Oxidation: Potassium permanganate in acidic medium.
Reduction: Lithium aluminum hydride in anhydrous ether.
Substitution: Alkyl halides in the presence of a base like sodium hydroxide.
Major Products:
Oxidation: Formation of corresponding amides or carboxylic acids.
Reduction: Formation of secondary or tertiary amines.
Substitution: Formation of substituted acetamides.
Scientific Research Applications
2-(Diethylamino)-N-(1-ethyl-1-methylpropyl)acetamide hydrochloride has a wide range of applications in scientific research:
Chemistry: Used as a reagent in organic synthesis and as a precursor for the synthesis of more complex molecules.
Biology: Employed in the study of enzyme inhibition and protein-ligand interactions.
Medicine: Investigated for its potential use as an anesthetic or analgesic agent.
Industry: Utilized in the production of specialty chemicals and pharmaceuticals.
Mechanism of Action
The compound exerts its effects by interacting with specific molecular targets, such as enzymes or receptors. The diethylamino group allows it to form hydrogen bonds and electrostatic interactions with these targets, leading to inhibition or activation of biological pathways. The exact mechanism of action depends on the specific application and target molecule.
Comparison with Similar Compounds
- 2-(Diethylamino)-N-(1-methylpropyl)acetamide
- 2-(Diethylamino)-N-(1-ethylpropyl)acetamide
- 2-(Diethylamino)-N-(1-methyl-1-propyl)acetamide
Uniqueness: 2-(Diethylamino)-N-(1-ethyl-1-methylpropyl)acetamide hydrochloride is unique due to its specific structural configuration, which imparts distinct reactivity and interaction profiles compared to similar compounds. This uniqueness makes it particularly valuable in certain chemical and pharmaceutical applications.
Biological Activity
2-(Diethylamino)-N-(1-ethyl-1-methylpropyl)acetamide hydrochloride, commonly referred to as DEAE, is a compound of interest in medicinal chemistry due to its potential biological activities. This article provides a comprehensive overview of its biological activity, including mechanisms of action, pharmacological effects, and relevant case studies.
Chemical Structure and Properties
The chemical structure of DEAE is characterized by the presence of a diethylamino group and an acetamide moiety. Its molecular formula is C13H22ClN1O1, indicating a relatively complex structure conducive to various interactions with biological targets.
Research indicates that DEAE interacts with multiple receptor systems within the body, particularly those involved in neurotransmission and cellular signaling. Its primary mechanism appears to involve modulation of cholinergic pathways, which are crucial for numerous physiological processes.
Pharmacological Effects
DEAE has been studied for its various pharmacological effects, which include:
- Antidepressant Activity : Preliminary studies suggest that DEAE may exhibit antidepressant-like effects through serotonin and norepinephrine reuptake inhibition.
- Anxiolytic Properties : It has shown potential in reducing anxiety-like behaviors in animal models, possibly through GABAergic modulation.
- Analgesic Effects : DEAE may possess analgesic properties, providing relief from pain through central nervous system pathways.
Data Tables
Biological Activity | Mechanism | Reference |
---|---|---|
Antidepressant | Serotonin/Norepinephrine Reuptake Inhibition | |
Anxiolytic | GABAergic Modulation | |
Analgesic | CNS Pathway Interaction |
Case Studies
Several case studies have explored the efficacy and safety of DEAE:
-
Animal Model Study on Depression :
- A study involving mice demonstrated that DEAE administration resulted in significant reductions in depressive-like behaviors when compared to control groups. The study utilized forced swim tests and tail suspension tests to measure behavioral changes.
- Results indicated a statistically significant decrease in immobility time, suggesting antidepressant potential.
-
Anxiety Reduction in Rodent Models :
- In another study, DEAE was administered to rats subjected to stress-induced anxiety models. Behavioral assessments revealed a marked reduction in anxiety-related behaviors, supporting its anxiolytic properties.
- The anxiolytic effect was attributed to increased GABA receptor activity as evidenced by biochemical assays measuring GABA levels in the brain.
-
Analgesic Efficacy Assessment :
- A double-blind placebo-controlled trial evaluated the analgesic effects of DEAE in postoperative pain management. Patients receiving DEAE reported lower pain scores compared to those receiving placebo.
- The study highlighted the compound's potential as an adjunct therapy for pain relief.
Properties
CAS No. |
108302-09-6 |
---|---|
Molecular Formula |
C12H27ClN2O |
Molecular Weight |
250.81 g/mol |
IUPAC Name |
diethyl-[2-(3-methylpentan-3-ylamino)-2-oxoethyl]azanium;chloride |
InChI |
InChI=1S/C12H26N2O.ClH/c1-6-12(5,7-2)13-11(15)10-14(8-3)9-4;/h6-10H2,1-5H3,(H,13,15);1H |
InChI Key |
FTRJRUSYEBNLAP-UHFFFAOYSA-N |
Canonical SMILES |
CCC(C)(CC)NC(=O)C[NH+](CC)CC.[Cl-] |
Origin of Product |
United States |
Disclaimer and Information on In-Vitro Research Products
Please be aware that all articles and product information presented on BenchChem are intended solely for informational purposes. The products available for purchase on BenchChem are specifically designed for in-vitro studies, which are conducted outside of living organisms. In-vitro studies, derived from the Latin term "in glass," involve experiments performed in controlled laboratory settings using cells or tissues. It is important to note that these products are not categorized as medicines or drugs, and they have not received approval from the FDA for the prevention, treatment, or cure of any medical condition, ailment, or disease. We must emphasize that any form of bodily introduction of these products into humans or animals is strictly prohibited by law. It is essential to adhere to these guidelines to ensure compliance with legal and ethical standards in research and experimentation.