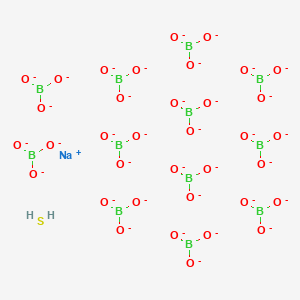
Sodium;sulfane;dodecaborate
- Click on QUICK INQUIRY to receive a quote from our team of experts.
- With the quality product at a COMPETITIVE price, you can focus more on your research.
Overview
Description
Sodium sulfane dodecaborate is a compound that features a unique arrangement of boron and hydrogen atoms It is known for its icosahedral structure, which consists of twelve boron atoms each bonded to a hydrogen atom
Preparation Methods
Synthetic Routes and Reaction Conditions: The synthesis of sodium sulfane dodecaborate typically involves the reaction of sodium borohydride with boron trifluoride etherate. The process can be summarized in two main steps:
Formation of Triborate Anion: [ 5 \text{NaBH}_4 + \text{BF}_3 \rightarrow 2 \text{NaB}_3\text{H}_8 + 3 \text{NaF} + 2 \text{H}_2 ]
Pyrolysis of Triborate: The triborate anion is then subjected to pyrolysis to yield the twelve-boron cluster as the sodium salt.
Industrial Production Methods: Industrial production of sodium sulfane dodecaborate involves heating a composite formed by introducing triethylammonium salt into the silicate matrix of sodium liquid glass at 200°C. This method results in the formation of nanoscale crystals of sodium sulfane dodecaborate on the surface of the silicate matrix .
Chemical Reactions Analysis
Types of Reactions: Sodium sulfane dodecaborate undergoes various chemical reactions, including:
Oxidation: The compound can be oxidized to form higher boron clusters.
Reduction: It can be reduced to form lower boron clusters.
Substitution: The hydrogen atoms in the compound can be replaced by halogens or other substituents.
Common Reagents and Conditions:
Oxidation: Hydrogen peroxide is commonly used for hydroxylation reactions.
Reduction: Sodium borohydride is used for reduction reactions.
Substitution: Halogenation reactions can be carried out using halogen gases under controlled conditions.
Major Products:
Oxidation Products: Higher boron clusters such as [B24H23]3−.
Reduction Products: Lower boron clusters.
Substitution Products: Halogenated derivatives of the compound.
Scientific Research Applications
Sodium sulfane dodecaborate has a wide range of applications in scientific research:
Chemistry: It is used in the synthesis of heat-resistant inorganic polymers and neutron-absorbing materials.
Medicine: Its derivatives are being studied for their therapeutic properties in various medical applications.
Industry: The compound is used in the production of nanomaterials and nanocomposites with unique properties.
Mechanism of Action
The mechanism of action of sodium sulfane dodecaborate involves its interaction with molecular targets and pathways. In BNCT, the compound accumulates in cancer cells and, upon neutron irradiation, releases high-energy particles that selectively destroy the tumor cells while sparing the surrounding healthy tissue .
Comparison with Similar Compounds
Sodium borocaptate (BSH): Used in BNCT, similar to sodium sulfane dodecaborate.
Boronophenylalanine (BPA): Another compound used in BNCT.
Uniqueness: Sodium sulfane dodecaborate is unique due to its icosahedral structure and stability. It offers advantages in terms of thermal and chemical stability, making it suitable for various high-temperature and high-stress applications .
Properties
Molecular Formula |
B12H2NaO36S-35 |
---|---|
Molecular Weight |
762.8 g/mol |
IUPAC Name |
sodium;sulfane;dodecaborate |
InChI |
InChI=1S/12BO3.Na.H2S/c12*2-1(3)4;;/h;;;;;;;;;;;;;1H2/q12*-3;+1; |
InChI Key |
SQKZYZYXPXGHER-UHFFFAOYSA-N |
Canonical SMILES |
B([O-])([O-])[O-].B([O-])([O-])[O-].B([O-])([O-])[O-].B([O-])([O-])[O-].B([O-])([O-])[O-].B([O-])([O-])[O-].B([O-])([O-])[O-].B([O-])([O-])[O-].B([O-])([O-])[O-].B([O-])([O-])[O-].B([O-])([O-])[O-].B([O-])([O-])[O-].[Na+].S |
Origin of Product |
United States |
Disclaimer and Information on In-Vitro Research Products
Please be aware that all articles and product information presented on BenchChem are intended solely for informational purposes. The products available for purchase on BenchChem are specifically designed for in-vitro studies, which are conducted outside of living organisms. In-vitro studies, derived from the Latin term "in glass," involve experiments performed in controlled laboratory settings using cells or tissues. It is important to note that these products are not categorized as medicines or drugs, and they have not received approval from the FDA for the prevention, treatment, or cure of any medical condition, ailment, or disease. We must emphasize that any form of bodily introduction of these products into humans or animals is strictly prohibited by law. It is essential to adhere to these guidelines to ensure compliance with legal and ethical standards in research and experimentation.