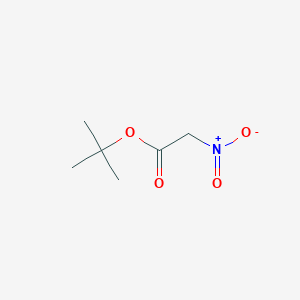
Tert-butyl nitroacetate
Overview
Description
Tert-butyl nitroacetate (CAS: Not explicitly provided; structurally analogous to ethyl nitroacetate, CAS 2483-56-9) is a nitro-substituted ester with the formula $ \text{C}6\text{H}{11}\text{NO}4 $. It is characterized by a tert-butyl ester group attached to a nitroacetate backbone ($ \text{NO}2\text{CH}2\text{COOR} $), which confers steric bulk and enhanced stability compared to smaller alkyl esters like methyl or ethyl nitroacetate. This compound is primarily utilized in organic synthesis as a versatile intermediate for cross-coupling reactions, cycloadditions, and the preparation of α-amino acids or heterocycles . Its reactivity stems from the highly acidic α-proton ($ \text{p}Ka \sim 5 $), enabling deprotonation to form a nucleophilic nitronate anion for Pd-catalyzed α-arylation or Michael additions .
Preparation Methods
Preparation Methods of Tert-butyl Nitroacetate
Nitration of Tert-butyl Alcohol with Nitrous Acid (HNO2)
One common approach to prepare tert-butyl nitro compounds involves reacting tert-butyl alcohol with nitrous acid. Since nitrous acid is unstable and decomposes rapidly, it is generated in situ by reacting sodium nitrite (NaNO2) with hydrochloric acid (HCl). This reaction is highly exothermic, requiring slow reagent addition and careful temperature control.
A recent continuous flow chemistry method has been developed to synthesize tert-butyl nitrite (a related nitro compound) with a high yield of 95% and a very short residence time of 1 minute. The flow process significantly improves reaction efficiency compared to traditional batch methods, which have cycle times of 12 to 24 hours. This method also enables metal-free stereoselective nitration of alkenes using tert-butyl nitrite as a nitrating agent, demonstrating its synthetic utility.
Reaction of Tert-butyl Alcohol with Aqueous Nitric Acid under Pressure
A patented method describes the preparation of nitro-tert-butyl alcohol and related nitro compounds by reacting tert-butyl alcohol or isobutene with aqueous nitric acid (30–85% by weight) at elevated temperatures (120–200 °C) and pressures sufficient to maintain the liquid phase. The reaction time ranges from 1 to 30 minutes, with typical pressures between 200 and 2000 psi.
The reaction mixture is heated in a pressure vessel or coil reactor, followed by cooling and fractional distillation to separate nitro-tert-butyl alcohol and α-nitro-isobutene. Typical yields for the nitro-alcohol and nitro-olefin products are about 70% combined, formed in roughly equimolar proportions. This method is notable for its continuous operation potential and relatively short reaction times.
Table 1: Reaction Conditions for Nitro-tert-butyl Alcohol Preparation
Parameter | Range/Value | Notes |
---|---|---|
Nitric acid concentration | 30–85% (wt) | Aqueous nitric acid |
Organic reactant | Tert-butyl alcohol or isobutene | 2–20 molar equivalents |
Temperature | 120–200 °C | Maintained below decomposition temp |
Pressure | 200–2000 psi (approx. 1.4–14 MPa) | To maintain liquid phase |
Reaction time | 1–30 minutes | Typically 1–10 minutes preferred |
Yield | ~70% combined products | Nitro-tert-butyl alcohol + α-nitro-isobutene |
Continuous Flow and Fixed Bed Reactor Techniques
The continuous flow synthesis approach for tert-butyl nitrite can be adapted for related nitro compounds, including this compound. Continuous air purging during the reaction ensures adequate oxygen supply, reducing reaction times dramatically from hours in batch to minutes in flow. This method is scalable and suitable for industrial production, offering improved safety and efficiency.
Comparative Analysis of Preparation Methods
Method | Key Reagents | Conditions | Yield/Outcome | Advantages | Limitations |
---|---|---|---|---|---|
In situ generation of HNO2 + tert-butyl alcohol | Sodium nitrite + HCl + tert-butyl alcohol | Room temp, exothermic, continuous flow | 95% yield (tert-butyl nitrite) | High yield, fast, continuous process | Requires careful control of exotherm |
Aqueous nitric acid + tert-butyl alcohol | 30–85% HNO3 + tert-butyl alcohol | 120–200 °C, 200–2000 psi, minutes | ~70% combined nitro products | Continuous operation, scalable | High pressure, elevated temp |
Catalytic oxidative esterification (related esters) | Iso-butane + acetic acid + catalysts | 40–75 °C, 0.6–2.0 MPa, hours | Up to ~30% yield (tert-butyl acetate) | Mild conditions, catalytic | Lower yield, longer reaction time |
Chemical Reactions Analysis
Types of Reactions: Tert-butyl nitroacetate undergoes various chemical reactions, including:
Oxidation: The nitro group can be oxidized to form nitro compounds with higher oxidation states.
Reduction: The nitro group can be reduced to an amine group (-NH₂) under appropriate conditions.
Substitution: The ester group can undergo nucleophilic substitution reactions, where the tert-butyl group is replaced by other nucleophiles.
Common Reagents and Conditions:
Oxidation: Common oxidizing agents include potassium permanganate (KMnO₄) and chromium trioxide (CrO₃).
Reduction: Reducing agents such as lithium aluminum hydride (LiAlH₄) or hydrogen gas (H₂) in the presence of a catalyst can be used.
Substitution: Nucleophiles like hydroxide ions (OH⁻) or alkoxides (RO⁻) can facilitate substitution reactions.
Major Products:
Oxidation: Formation of nitro compounds with higher oxidation states.
Reduction: Formation of tert-butyl aminoacetate.
Substitution: Formation of various esters depending on the nucleophile used.
Scientific Research Applications
Organic Synthesis
Tert-butyl nitroacetate serves as a significant reagent in organic chemistry, particularly in the synthesis of complex molecules. Its unique structural properties allow it to participate in various chemical reactions:
- Nitration Reactions : this compound can be employed in the nitration of aliphatic and aromatic compounds. It acts as a source of nitro groups, facilitating the introduction of nitro functionalities into organic substrates. This is crucial for synthesizing compounds with specific electronic and steric properties .
- Diazotization : The compound is also used in diazotization reactions, where it can generate diazonium salts from primary amines. These intermediates are essential for further transformations in organic synthesis, such as coupling reactions to form azo compounds .
- Nitrosylation : this compound has been explored for its ability to undergo nitrosylation reactions, where it can introduce nitroso groups into various substrates. This process is valuable for creating compounds with unique biological activities .
Pharmaceutical Applications
The pharmaceutical industry has shown interest in this compound due to its potential as an intermediate in drug synthesis:
- Drug Development : The compound's ability to introduce functional groups makes it a candidate for developing new pharmaceuticals. Its derivatives may exhibit enhanced biological activity or improved pharmacokinetic properties .
- Bioactive Compounds : Research indicates that this compound derivatives could serve as bioactive agents. Studies have demonstrated that modifications of the compound can lead to substances with potential therapeutic effects .
Case Studies
Several case studies highlight the applications of this compound in research:
- Nitro-Nitratosation of Alkenes : A study demonstrated that this compound could mediate the nitration of internal alkenes under oxygen atmospheres, yielding products with nitrato groups. This reaction showcases its utility in synthesizing complex organic molecules with multiple functional groups .
- Synthesis of Nitro Compounds : In another study, this compound was utilized to synthesize various nitro compounds via straightforward nitration methods. The resulting products exhibited promising chemical properties for further applications in material science and pharmaceuticals .
Mechanism of Action
The mechanism of action of tert-butyl nitroacetate involves its reactivity towards various chemical reagents. The nitro group can participate in electron-withdrawing interactions, making the compound susceptible to nucleophilic attack. The ester group can undergo hydrolysis or transesterification reactions, leading to the formation of different products depending on the reaction conditions .
Comparison with Similar Compounds
Structural and Physicochemical Properties
The table below compares tert-butyl nitroacetate with analogous nitroacetates and related esters:
Reactivity in Cross-Coupling Reactions
- This compound : Demonstrates moderate reactivity in Pd-catalyzed α-arylation due to steric hindrance from the tert-butyl group, which slows transmetallation steps. Optimal conditions require 2.5 mol% $ \text{Pd}2\text{dba}3 $, 10 mol% t-BuXPhos ligand, and $ \text{CsHCO}_3 $ in toluene at 75°C, yielding 52–96% isolated products .
- Ethyl nitroacetate: More reactive in the same reactions due to reduced steric bulk, achieving higher yields (e.g., 96% with electron-poor aryl bromides). However, it forms less stable anions in polar solvents, necessitating non-polar media .
- Methyl nitroacetate: Limited use in cross-coupling due to competing side reactions and lower thermal stability .
Stability and Handling
- This compound: Exhibits superior thermal and hydrolytic stability compared to ethyl/methyl analogs, attributed to steric protection of the ester group. No significant toxicity data are available, but handling precautions (gloves, eye protection) are recommended .
- Ethyl nitroacetate : Requires storage at low temperatures to prevent decomposition. In ruminal studies, it reduces methane production by 30–50% but inhibits volatile fatty acid synthesis .
- Methyl nitroacetate : Classified as harmful if swallowed; strict safety protocols (ventilation, PPE) are mandatory .
Limitations and Challenges
- This compound: Limited solubility in polar solvents restricts its use in aqueous or protic media. Steric effects also hinder reactions with bulky electrophiles (e.g., ortho-substituted aryl bromides) .
- Ethyl nitroacetate : Generates insoluble nitronate anions with strong bases (e.g., $ \text{Cs}2\text{CO}3 $), complicating purification .
- Methyl nitroacetate : Narrow synthetic utility due to competing ester hydrolysis or nitro group reduction .
Q & A
Basic Research Questions
Q. What are the common synthetic routes for preparing tert-butyl nitroacetate derivatives, and how do reaction conditions influence product purity?
- Methodological Answer : this compound derivatives are typically synthesized via nitro-Mannich reactions or Michael additions. For example, nitroacetic esters react with aldehydes under TiCl4 and amine catalysis to form intermediates like substituted nitroacrylates . Ethyl nitroacetate can also undergo cycloaddition with organic azides to yield isoxazole derivatives, though excess n-butylamine may lead to butylamide byproducts . Key factors include solvent choice (e.g., anhydrous THF), temperature control (0–25°C), and catalyst stoichiometry to minimize side reactions. Purity is often assessed via HPLC or <sup>13</sup>C NMR to confirm ester group integrity.
Q. How can researchers characterize this compound derivatives to confirm structural identity?
- Methodological Answer : Structural elucidation relies on spectroscopic techniques:
- NMR : <sup>1</sup>H NMR detects nitro group protons (δ 4.5–5.0 ppm) and tert-butyl protons (δ 1.2–1.4 ppm). <sup>13</sup>C NMR identifies carbonyl carbons (δ 165–170 ppm) .
- Mass Spectrometry : High-resolution MS (HRMS) confirms molecular formulas (e.g., C12H13N3O4 for tert-butyl 2-cyano-2-(5-nitropyridin-2-yl)acetate) .
- X-ray Crystallography : Resolves stereochemistry in cycloadducts like isoxazoles .
Q. What are the primary applications of this compound in medicinal chemistry?
- Methodological Answer : Derivatives are intermediates for bioactive molecules. For instance:
- Anticancer Agents : Nitropyridyl-acetate derivatives are screened against kinase targets using cell viability assays (e.g., MTT) .
- Antimicrobials : Nitroacetate Schiff bases are tested via microdilution assays against Gram-positive bacteria .
- Peptide Mimetics : Cα,α-dialkylated nitroacetates serve as β-strand mimics in amyloid fibril inhibition studies .
Advanced Research Questions
Q. How do stereochemical outcomes vary in this compound-mediated cycloadditions, and what strategies mitigate undesired diastereomers?
- Methodological Answer : Cycloadditions with dipolarophiles (e.g., azides) often yield isoxazoles with stereocenter formation at the nitro-bearing carbon. Diastereoselectivity is influenced by:
- Substrate Electronics : Electron-withdrawing groups (e.g., pyridyl) enhance regioselectivity .
- Solvent Polarity : Polar aprotic solvents (e.g., DMF) favor transition-state stabilization .
- Catalytic Asymmetry : Chiral amines (e.g., cinchona alkaloids) can induce enantioselectivity, though tert-butyl esters may sterically hinder catalyst binding .
Q. What experimental approaches resolve contradictions in reported yields for nitroacetate-Schiff base condensations?
- Methodological Answer : Discrepancies arise from:
- Base Sensitivity : Tert-butyl esters hydrolyze under basic conditions, requiring pH monitoring (pH 7–8) .
- Intermediate Trapping : Use quenching agents (e.g., acetic acid) to stabilize reactive intermediates like enamines .
- Byproduct Analysis : LC-MS identifies competing pathways (e.g., over-alkylation) .
Q. How does this compound’s stability under thermal or photolytic conditions impact reaction design?
- Methodological Answer : Thermolysis of nitroacetates generates fulminic acid, a hazardous byproduct . Mitigation strategies include:
- Temperature Control : Reactions below 60°C to prevent decomposition .
- Light Exclusion : Amber glassware or darkroom setups to avoid photolytic cleavage .
- Real-Time Monitoring : In situ IR spectroscopy tracks nitro group degradation (νNO2 at ~1550 cm<sup>−1</sup>) .
Q. What advanced catalytic systems improve enantioselectivity in this compound-based asymmetric syntheses?
Properties
IUPAC Name |
tert-butyl 2-nitroacetate | |
---|---|---|
Details | Computed by LexiChem 2.6.6 (PubChem release 2019.06.18) | |
Source | PubChem | |
URL | https://pubchem.ncbi.nlm.nih.gov | |
Description | Data deposited in or computed by PubChem | |
InChI |
InChI=1S/C6H11NO4/c1-6(2,3)11-5(8)4-7(9)10/h4H2,1-3H3 | |
Details | Computed by InChI 1.0.5 (PubChem release 2019.06.18) | |
Source | PubChem | |
URL | https://pubchem.ncbi.nlm.nih.gov | |
Description | Data deposited in or computed by PubChem | |
InChI Key |
QMQBQFMIOXUDNV-UHFFFAOYSA-N | |
Details | Computed by InChI 1.0.5 (PubChem release 2019.06.18) | |
Source | PubChem | |
URL | https://pubchem.ncbi.nlm.nih.gov | |
Description | Data deposited in or computed by PubChem | |
Canonical SMILES |
CC(C)(C)OC(=O)C[N+](=O)[O-] | |
Details | Computed by OEChem 2.1.5 (PubChem release 2019.06.18) | |
Source | PubChem | |
URL | https://pubchem.ncbi.nlm.nih.gov | |
Description | Data deposited in or computed by PubChem | |
Molecular Formula |
C6H11NO4 | |
Details | Computed by PubChem 2.1 (PubChem release 2019.06.18) | |
Source | PubChem | |
URL | https://pubchem.ncbi.nlm.nih.gov | |
Description | Data deposited in or computed by PubChem | |
Molecular Weight |
161.16 g/mol | |
Details | Computed by PubChem 2.1 (PubChem release 2021.05.07) | |
Source | PubChem | |
URL | https://pubchem.ncbi.nlm.nih.gov | |
Description | Data deposited in or computed by PubChem | |
Disclaimer and Information on In-Vitro Research Products
Please be aware that all articles and product information presented on BenchChem are intended solely for informational purposes. The products available for purchase on BenchChem are specifically designed for in-vitro studies, which are conducted outside of living organisms. In-vitro studies, derived from the Latin term "in glass," involve experiments performed in controlled laboratory settings using cells or tissues. It is important to note that these products are not categorized as medicines or drugs, and they have not received approval from the FDA for the prevention, treatment, or cure of any medical condition, ailment, or disease. We must emphasize that any form of bodily introduction of these products into humans or animals is strictly prohibited by law. It is essential to adhere to these guidelines to ensure compliance with legal and ethical standards in research and experimentation.