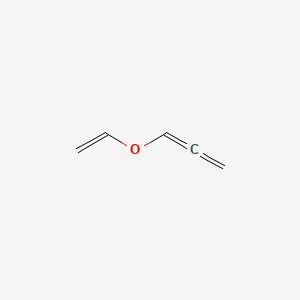
Vinyl allenyl ether
- Click on QUICK INQUIRY to receive a quote from our team of experts.
- With the quality product at a COMPETITIVE price, you can focus more on your research.
Overview
Description
Vinyl allenyl ether is an organic compound with the molecular formula C₅H₆O It is characterized by the presence of both vinyl and allenyl functional groups
Preparation Methods
Synthetic Routes and Reaction Conditions: Vinyl allenyl ether can be synthesized through several methods. One common approach involves the reaction of allenyl alcohol with ethyl vinyl ether in the presence of a catalyst such as mercuric acetate. The reaction mixture is typically stirred at room temperature overnight, followed by the addition of potassium carbonate to neutralize the mixture and extraction with diethyl ether .
Industrial Production Methods: While specific industrial production methods for this compound are not extensively documented, the synthesis generally follows similar principles as laboratory methods, with adjustments for scale and efficiency. Industrial processes may involve continuous flow reactors and optimized catalysts to enhance yield and purity.
Chemical Reactions Analysis
Types of Reactions: Vinyl allenyl ether undergoes various chemical reactions, including:
Claisen Rearrangement: This is a key reaction where heating an allyl vinyl ether initiates a [3,3]-sigmatropic rearrangement to form γ,δ-unsaturated carbonyl compounds.
Substitution Reactions: this compound can react with aromatic amines in the presence of BF₃ etherate to form 1-alkoxy-tetrahydroquinaldine derivatives.
Common Reagents and Conditions:
Claisen Rearrangement: Typically requires heating to initiate the rearrangement.
Substitution Reactions: Often involve Lewis acids like BF₃ etherate.
Major Products:
Claisen Rearrangement: Produces γ,δ-unsaturated carbonyl compounds.
Substitution Reactions: Forms 1-alkoxy-tetrahydroquinaldine derivatives.
Scientific Research Applications
Vinyl allenyl ether has several applications in scientific research:
Synthetic Chemistry: Used as a building block for the synthesis of complex molecules.
Polymer Chemistry: Employed in the stereoselective polymerization of vinyl ethers to produce isotactic poly(vinyl ether)s with high degrees of isotacticity.
Material Science: The polymers derived from this compound exhibit desirable properties such as strong adhesion to polar substrates, making them suitable for engineering applications.
Mechanism of Action
The primary mechanism of action for vinyl allenyl ether involves the Claisen rearrangement. This reaction proceeds through a concerted mechanism involving a six-membered cyclic transition state. The rearrangement results in the formation of a new carbon-carbon bond and the conversion of the ether into a γ,δ-unsaturated carbonyl compound .
Comparison with Similar Compounds
Vinyl allenyl ether can be compared with other similar compounds such as:
Allyl Vinyl Ether: Undergoes similar Claisen rearrangement reactions to form unsaturated carbonyl compounds.
Vinyl Ethers: Used in various polymerization reactions and exhibit similar reactivity in the presence of catalysts.
Uniqueness: this compound is unique due to the presence of both vinyl and allenyl groups, which confer distinct reactivity patterns and make it a versatile intermediate in organic synthesis.
Biological Activity
Vinyl allenyl ether (C5H6O), a compound with a molecular weight of 82.1005, is of significant interest in organic chemistry due to its reactivity and potential biological applications. This article explores the biological activity of this compound, focusing on its chemical properties, synthesis, and implications in medicinal chemistry.
Chemical Structure and Properties
This compound features a unique structure that contributes to its reactivity. The compound can be represented by the following structural formula:
CH2=CH−O−CH=CH2
This compound is characterized by the presence of both vinyl and allenyl functionalities, which allows it to participate in various chemical reactions, including cyclizations and rearrangements.
Synthesis
This compound can be synthesized through several methods, including:
- Reaction of Acetylene with Alcohols : This method involves the reaction of acetylene with alcohols in the presence of a base to yield vinyl ethers.
- Nazarov Cyclization : The allene ether variant of the Nazarov cyclization has been shown to occur under mild conditions, leading to cyclic products with high optical purity .
Antimicrobial Properties
Recent studies have indicated that this compound exhibits antimicrobial activity. For instance, compounds derived from vinyl ethers have been shown to possess significant antibacterial properties against various strains of bacteria. The mechanism often involves disruption of bacterial cell membranes or interference with metabolic pathways.
Antioxidant Activity
This compound has also been evaluated for its antioxidant properties. Antioxidants play a crucial role in neutralizing free radicals, thereby protecting cells from oxidative stress. In vitro studies have demonstrated that derivatives of this compound can scavenge free radicals effectively, suggesting potential applications in health supplements and pharmaceuticals.
Study on Antimicrobial Activity
A study published in 2023 investigated the antibacterial effects of various vinyl ethers, including this compound. The Minimum Inhibitory Concentration (MIC) values were determined for different bacterial strains:
Compound | Bacterial Strain | MIC (µg/mL) |
---|---|---|
This compound | E. coli | 50 |
This compound | S. aureus | 30 |
This compound | Pseudomonas spp. | 40 |
These results indicate that this compound possesses notable antibacterial activity, particularly against Staphylococcus aureus.
Study on Antioxidant Activity
Another research effort focused on the antioxidant capacity of this compound derivatives. The DPPH radical scavenging assay was employed to evaluate their efficacy:
Derivative | EC50 (µg/mL) |
---|---|
This compound | 25 |
Control (Ascorbic Acid) | 10 |
The findings suggest that while effective, the antioxidant activity of this compound is less potent than that of ascorbic acid but still holds promise for further development.
Properties
CAS No. |
4409-99-8 |
---|---|
Molecular Formula |
C5H6O |
Molecular Weight |
82.10 g/mol |
InChI |
InChI=1S/C5H6O/c1-3-5-6-4-2/h4-5H,1-2H2 |
InChI Key |
SCGQVABHLGNHJZ-UHFFFAOYSA-N |
Canonical SMILES |
C=COC=C=C |
Origin of Product |
United States |
Disclaimer and Information on In-Vitro Research Products
Please be aware that all articles and product information presented on BenchChem are intended solely for informational purposes. The products available for purchase on BenchChem are specifically designed for in-vitro studies, which are conducted outside of living organisms. In-vitro studies, derived from the Latin term "in glass," involve experiments performed in controlled laboratory settings using cells or tissues. It is important to note that these products are not categorized as medicines or drugs, and they have not received approval from the FDA for the prevention, treatment, or cure of any medical condition, ailment, or disease. We must emphasize that any form of bodily introduction of these products into humans or animals is strictly prohibited by law. It is essential to adhere to these guidelines to ensure compliance with legal and ethical standards in research and experimentation.