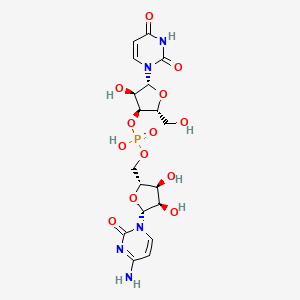
Uridylyl-(3'-5')-cytidine
- Click on QUICK INQUIRY to receive a quote from our team of experts.
- With the quality product at a COMPETITIVE price, you can focus more on your research.
Overview
Description
Uridylyl-(3’-5’)-cytidine is a nucleotide analog composed of uridine and cytidine linked by a phosphodiester bond between the 3’ hydroxyl group of uridine and the 5’ hydroxyl group of cytidine. This compound is of significant interest in biochemical and molecular biology research due to its role in RNA synthesis and function.
Preparation Methods
Synthetic Routes and Reaction Conditions: The synthesis of uridylyl-(3’-5’)-cytidine typically involves the chemical synthesis of oligonucleotides. The process begins with the cleavage of the 5’-trityl group by brief treatment with dichloroacetic acid dissolved in dichloromethane. The monomer activated with tetrazole is then coupled to the available 5’-hydroxyl, resulting in a phosphite linkage . This method is commonly used in the synthesis of DNA and RNA oligonucleotides, allowing for the creation of nucleotide sequences with defined chemical structures.
Industrial Production Methods: Industrial production of uridylyl-(3’-5’)-cytidine follows similar synthetic routes but on a larger scale. Automated high-throughput custom oligonucleotide synthesis technologies have made it possible to produce large quantities of nucleotide analogs efficiently .
Chemical Reactions Analysis
Types of Reactions: Uridylyl-(3’-5’)-cytidine undergoes various chemical reactions, including hydrolysis, catalyzed by metal ions such as magnesium and zinc. These reactions can be promoted by buffers like imidazole, HEPES, and triethanolamine . The compound can also undergo cleavage and isomerization in the presence of amine buffers such as morpholine, 4-hydroxypiperidine, and piperidine .
Common Reagents and Conditions: Common reagents used in the reactions of uridylyl-(3’-5’)-cytidine include metal ions (e.g., magnesium, zinc), buffers (e.g., imidazole, HEPES), and amine buffers (e.g., morpholine, 4-hydroxypiperidine) . These reactions typically occur under aqueous conditions, often with the addition of organic solvents like DMSO to modulate the reaction environment .
Major Products Formed: The major products formed from the reactions of uridylyl-(3’-5’)-cytidine include cleaved nucleotide fragments and isomerized forms of the compound. These products are of interest in studying the mechanisms of RNA cleavage and isomerization .
Scientific Research Applications
Uridylyl-(3’-5’)-cytidine has numerous applications in scientific research, particularly in the fields of chemistry, biology, and medicine. It is used as a model compound to study RNA cleavage and isomerization mechanisms . Additionally, it plays a role in understanding the catalytic activities of metal ions and buffers in RNA reactions . In medicine, nucleotide analogs like uridylyl-(3’-5’)-cytidine are explored for their potential therapeutic applications, including antiviral and anticancer treatments.
Mechanism of Action
The mechanism of action of uridylyl-(3’-5’)-cytidine involves its incorporation into RNA molecules, where it can influence RNA stability and function. The compound can be cleaved by metal-ion-promoted hydrolysis, with metal ions such as magnesium and zinc playing a crucial role in the catalytic process . The cleavage and isomerization reactions are influenced by the coordination of metal ions to the anionic phosphodiester and the participation of buffer constituents .
Comparison with Similar Compounds
Uridylyl-(3’-5’)-cytidine can be compared to other nucleotide analogs such as uridylyl-(3’-5’)-uridine and 2-hydroxypropyl 4-nitrophenyl phosphate. While uridylyl-(3’-5’)-uridine shares a similar structure, it differs in its base composition, which can affect its reactivity and interactions with metal ions and buffers . 2-hydroxypropyl 4-nitrophenyl phosphate is an activated RNA model compound used to study RNA cleavage mechanisms, providing insights into the catalytic activities of metal ions and buffers .
Conclusion
Uridylyl-(3’-5’)-cytidine is a valuable compound in biochemical and molecular biology research, offering insights into RNA synthesis, cleavage, and isomerization mechanisms. Its synthesis, reactions, and applications make it a crucial tool for understanding the catalytic activities of metal ions and buffers in RNA reactions.
Properties
CAS No. |
3013-97-6 |
---|---|
Molecular Formula |
C18H24N5O13P |
Molecular Weight |
549.4 g/mol |
IUPAC Name |
[(2R,3S,4R,5R)-5-(4-amino-2-oxopyrimidin-1-yl)-3,4-dihydroxyoxolan-2-yl]methyl [(2R,3S,4R,5R)-5-(2,4-dioxopyrimidin-1-yl)-4-hydroxy-2-(hydroxymethyl)oxolan-3-yl] hydrogen phosphate |
InChI |
InChI=1S/C18H24N5O13P/c19-9-1-3-22(17(29)20-9)15-12(27)11(26)8(35-15)6-33-37(31,32)36-14-7(5-24)34-16(13(14)28)23-4-2-10(25)21-18(23)30/h1-4,7-8,11-16,24,26-28H,5-6H2,(H,31,32)(H2,19,20,29)(H,21,25,30)/t7-,8-,11-,12-,13-,14-,15-,16-/m1/s1 |
InChI Key |
LENQVXALZPDAFB-NCOIDOBVSA-N |
Isomeric SMILES |
C1=CN(C(=O)N=C1N)[C@H]2[C@@H]([C@@H]([C@H](O2)COP(=O)(O)O[C@@H]3[C@H](O[C@H]([C@@H]3O)N4C=CC(=O)NC4=O)CO)O)O |
Canonical SMILES |
C1=CN(C(=O)N=C1N)C2C(C(C(O2)COP(=O)(O)OC3C(OC(C3O)N4C=CC(=O)NC4=O)CO)O)O |
Origin of Product |
United States |
Disclaimer and Information on In-Vitro Research Products
Please be aware that all articles and product information presented on BenchChem are intended solely for informational purposes. The products available for purchase on BenchChem are specifically designed for in-vitro studies, which are conducted outside of living organisms. In-vitro studies, derived from the Latin term "in glass," involve experiments performed in controlled laboratory settings using cells or tissues. It is important to note that these products are not categorized as medicines or drugs, and they have not received approval from the FDA for the prevention, treatment, or cure of any medical condition, ailment, or disease. We must emphasize that any form of bodily introduction of these products into humans or animals is strictly prohibited by law. It is essential to adhere to these guidelines to ensure compliance with legal and ethical standards in research and experimentation.