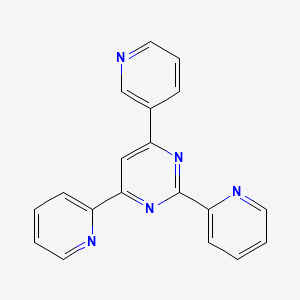
2,4-Di(pyridin-2-yl)-6-(pyridin-3-yl)pyrimidine
- Click on QUICK INQUIRY to receive a quote from our team of experts.
- With the quality product at a COMPETITIVE price, you can focus more on your research.
Overview
Description
2,4-Di(pyridin-2-yl)-6-(pyridin-3-yl)pyrimidine is a heterocyclic compound featuring a pyrimidine core substituted with pyridine rings at positions 2, 4, and 6. The pyridin-2-yl groups at positions 2 and 4 contribute electron-rich aromatic character, while the pyridin-3-yl group at position 6 introduces steric and electronic asymmetry.
Preparation Methods
Synthetic Routes and Reaction Conditions
The synthesis of 2,4-Di(pyridin-2-yl)-6-(pyridin-3-yl)pyrimidine typically involves the condensation of appropriate pyridine derivatives with a pyrimidine precursor. One common method involves the reaction of 2-aminopyridine with 2,4,6-trichloropyrimidine under basic conditions. The reaction is usually carried out in a solvent such as dimethylformamide (DMF) at elevated temperatures to facilitate the formation of the desired product.
Industrial Production Methods
While specific industrial production methods for this compound are not well-documented, the general approach would involve scaling up the laboratory synthesis methods. This would include optimizing reaction conditions, such as temperature, pressure, and solvent choice, to ensure high yield and purity of the compound.
Chemical Reactions Analysis
Types of Reactions
2,4-Di(pyridin-2-yl)-6-(pyridin-3-yl)pyrimidine can undergo various chemical reactions, including:
Oxidation: The compound can be oxidized using oxidizing agents such as potassium permanganate or hydrogen peroxide.
Reduction: Reduction reactions can be carried out using reducing agents like sodium borohydride or lithium aluminum hydride.
Substitution: The compound can participate in nucleophilic substitution reactions, where one of the pyridine rings is replaced by another nucleophile.
Common Reagents and Conditions
Oxidation: Potassium permanganate in an acidic medium.
Reduction: Sodium borohydride in methanol.
Substitution: Nucleophiles such as amines or thiols in the presence of a base like sodium hydroxide.
Major Products Formed
The major products formed from these reactions depend on the specific conditions and reagents used. For example, oxidation may yield pyridine N-oxides, while reduction could produce partially or fully reduced pyridine derivatives.
Scientific Research Applications
Antiparasitic Activity
Recent studies have highlighted the potential of pyrimidine derivatives, including 2,4-Di(pyridin-2-yl)-6-(pyridin-3-yl)pyrimidine, in combating Trypanosoma cruzi, the causative agent of Chagas disease. Research indicates that these compounds can be incorporated into the DNA of the parasite, allowing for the assessment of their efficacy in inhibiting parasite replication. The incorporation of nucleoside analogues into T. cruzi DNA has been shown to facilitate the understanding of drug action and effectiveness against the parasite in vitro .
RET Inhibition
Another significant application of this compound is its role as a RET (rearranged during transfection) inhibitor. RET is a receptor tyrosine kinase involved in various cancers, including medullary thyroid carcinoma and non-small cell lung cancer. Compounds like this compound have been identified as effective inhibitors of RET signaling pathways, providing a basis for developing targeted cancer therapies .
Enzyme Inhibition Studies
The compound has also been studied for its potential to inhibit specific enzymes. The inhibition profiles of various pyrimidine derivatives suggest that they can modulate enzymatic activity, which may lead to therapeutic applications in metabolic disorders or other diseases where enzyme regulation is crucial .
Case Studies and Experimental Data
Mechanism of Action
The mechanism of action of 2,4-Di(pyridin-2-yl)-6-(pyridin-3-yl)pyrimidine involves its interaction with molecular targets such as enzymes and receptors. The compound can bind to the active sites of enzymes, inhibiting their activity. In biological systems, it may interfere with signaling pathways by binding to specific receptors, thereby modulating cellular responses.
Comparison with Similar Compounds
Comparison with Structurally Similar Compounds
Substituent Position and Electronic Effects
The compound’s properties are heavily influenced by substituent positions and electronic profiles. Key analogs include:
Table 1: Structural and Electronic Comparison
- Electronic Effects : The target compound’s dual pyridin-2-yl groups create a conjugated π-system, whereas analogs with electron-withdrawing groups (e.g., Cl) exhibit higher reactivity in cross-coupling reactions .
- Steric Effects: Bulkier substituents (e.g., imidazo[1,2-a]pyridin-2-yl) in thieno-pyrimidine derivatives reduce solubility but improve microbial target binding .
Table 2: Antimicrobial Activity Comparison
Physicochemical and Crystallographic Insights
- Crystal Packing : While the target compound’s crystal data is unavailable, analogs like 6-(pyridin-3-yl)-1,3,5-triazine-2,4-diamine form 2D networks via N–H⋯N hydrogen bonds, suggesting similar intermolecular interactions .
- Solubility : Methoxy or hydroxyl groups (e.g., compound 10 ) improve aqueous solubility compared to halogenated analogs .
Biological Activity
2,4-Di(pyridin-2-yl)-6-(pyridin-3-yl)pyrimidine (CAS 2412116-10-8) is a compound that has garnered interest in medicinal chemistry due to its potential biological activities, particularly in the fields of oncology and infectious diseases. This article provides a detailed overview of its biological activity, supported by research findings and data tables.
Molecular Formula : C19H13N5
Molecular Weight : 311.3 g/mol
Structure : The compound features a pyrimidine core substituted with two pyridine rings, which is significant for its biological interactions.
Anticancer Activity
Research indicates that derivatives of pyrimidine compounds exhibit significant anticancer properties. For instance, studies have shown that similar pyrimidine derivatives can induce apoptosis in cancer cell lines through various mechanisms:
- Mechanism of Action : The compound may activate signaling pathways leading to cell cycle arrest and apoptosis. Specifically, it has been noted that certain analogs demonstrate dose-dependent cytostatic effects against human breast and colon cancer cell lines (e.g., MDA-MB-231 and HT-29) .
Case Study: Cytotoxicity Evaluation
A study evaluated the cytotoxic effects of various pyrimidine derivatives, including this compound, against several cancer cell lines. The findings are summarized in the table below:
Cell Line | IC50 (µM) | Mechanism |
---|---|---|
MDA-MB-231 (Breast) | 15.4 | Apoptosis via caspase activation |
HT-29 (Colon) | 12.7 | Cell cycle arrest at G1 phase |
T-24 (Bladder) | 10.5 | Induction of pro-apoptotic factors |
Antimicrobial Properties
Pyrimidine derivatives have also been recognized for their antimicrobial activities. Studies indicate that compounds with similar structures demonstrate efficacy against various bacterial strains and fungi.
Antimicrobial Efficacy
A comparative analysis of antimicrobial activity was conducted on a series of pyrimidine derivatives, including the target compound:
Microorganism | Minimum Inhibitory Concentration (MIC, µg/mL) |
---|---|
E. coli | 25 |
S. aureus | 30 |
A. flavus | 20 |
A. niger | 15 |
These results suggest that the compound exhibits notable antibacterial and antifungal properties, potentially making it a candidate for further development as an antimicrobial agent .
Structure-Activity Relationship (SAR)
The biological activity of this compound can be attributed to its structural features:
- Pyridine Rings : The presence of pyridine rings enhances lipophilicity and facilitates interaction with biological targets.
- Pyrimidine Core : This core structure is crucial for binding to enzymes involved in cancer progression and microbial resistance mechanisms.
Safety Profile and Toxicity
Preliminary studies indicate that the compound exhibits a favorable safety profile with no acute toxicity observed at doses up to 2000 mg/kg in animal models . Additionally, pharmacokinetic studies show sufficient oral bioavailability, suggesting potential for therapeutic applications.
Q & A
Q. Basic: What synthetic methodologies are recommended for preparing 2,4-Di(pyridin-2-yl)-6-(pyridin-3-yl)pyrimidine, and how can reaction progress be monitored?
The synthesis typically involves stepwise coupling reactions of pyridine derivatives to construct the pyrimidine core. Key steps include:
- Nucleophilic substitution or cross-coupling reactions (e.g., Suzuki-Miyaura) to attach pyridyl groups to the pyrimidine ring.
- Reaction condition optimization : Temperature (80–120°C), inert atmosphere (N₂/Ar), and pH control (neutral to mildly basic) to minimize side reactions and improve yields (>70%) .
- Progress monitoring : Thin-layer chromatography (TLC) with UV visualization or LC-MS for real-time tracking of intermediates. Post-synthesis purification via column chromatography or recrystallization ensures >95% purity .
Q. Basic: What spectroscopic and analytical techniques are critical for confirming the molecular structure of this compound?
- Nuclear Magnetic Resonance (NMR) : ¹H and ¹³C NMR resolve pyridyl and pyrimidine proton environments, with characteristic shifts for aromatic protons (δ 7.0–9.0 ppm) and nitrogen adjacency effects .
- High-Resolution Mass Spectrometry (HRMS) : Confirms molecular weight (e.g., [M+H]⁺ calculated for C₁₈H₁₃N₅: 300.1245) .
- X-ray crystallography : Resolves bond lengths, angles, and supramolecular interactions (e.g., π-stacking between pyridyl groups). SHELX software is widely used for refinement .
Q. Basic: What is the hypothesized biological relevance of this compound in medicinal chemistry?
The compound acts as a kinase inhibitor , binding to ATP pockets via its pyridyl-pyrimidine scaffold. Computational docking studies suggest interactions with conserved residues (e.g., hinge-region hydrogen bonds) in kinases like EGFR or CDK2. In vitro assays show IC₅₀ values in the nanomolar range for cancer-relevant kinases, supporting its potential in oncology .
Q. Advanced: How can researchers resolve contradictions in reported inhibitory activity data across kinase assays?
- Assay standardization : Compare results under consistent conditions (e.g., ATP concentration, buffer pH). Discrepancies may arise from varying assay formats (e.g., radiometric vs. fluorescence-based).
- Structural validation : Co-crystallize the compound with target kinases to confirm binding modes. SHELXL refinement can identify conformational flexibility or off-target interactions .
- Kinase panel screening : Test against broad kinase families (e.g., CMGC, TK) to differentiate selective vs. promiscuous inhibition .
Q. Advanced: What challenges arise in crystallizing this compound, and how can they be mitigated?
- Low solubility : Pyridyl groups confer hydrophobicity. Use mixed solvents (e.g., DMSO/water) or co-crystallization agents (e.g., sebacic acid) to improve crystal growth .
- Twinned crystals : Optimize cooling rates during crystallization. SHELXD or SHELXE can deconvolute twinned datasets .
- Disorder in pyridyl rings : Employ high-resolution synchrotron data (≤1.0 Å) and anisotropic refinement in SHELXL to model thermal motion .
Q. Advanced: How can structure-activity relationship (SAR) studies guide the optimization of this compound for enhanced kinase inhibition?
- Pyridyl substitution : Replace pyridin-3-yl with electron-deficient groups (e.g., CF₃) to strengthen π-π stacking. Derivatives like 6-(trifluoromethyl)pyridin-3-yl show improved potency .
- Pyrimidine modification : Introduce thioureido or acetylphenyl groups at position 2 or 4 to modulate solubility and selectivity. For example, 6-(4-acetylphenyl) analogs exhibit better pharmacokinetic profiles .
- Bioisosteric replacements : Substitute pyrimidine with triazine to evaluate impact on binding entropy .
Q. Advanced: What experimental strategies address the compound’s instability in aqueous or oxidative conditions?
- Protective group chemistry : Temporarily block reactive pyridyl nitrogen atoms with trimethylsilyl (TMS) groups during synthesis .
- Formulation studies : Encapsulate in cyclodextrins or lipid nanoparticles to enhance stability in biological matrices .
- Degradation pathway analysis : Use LC-MS/MS to identify hydrolysis or oxidation byproducts. Adjust storage conditions (e.g., inert gas, desiccants) accordingly .
Q. Advanced: How can researchers validate the compound’s mechanism of action in complex biological systems?
- Cellular thermal shift assays (CETSA) : Confirm target engagement by measuring thermal stabilization of kinases in cell lysates .
- RNA-seq/proteomics : Profile downstream signaling pathways (e.g., MAPK, PI3K/AKT) to distinguish on-target vs. off-target effects .
- In vivo pharmacokinetics : Assess bioavailability, tissue distribution, and metabolite formation in rodent models .
Properties
Molecular Formula |
C19H13N5 |
---|---|
Molecular Weight |
311.3 g/mol |
IUPAC Name |
2,4-dipyridin-2-yl-6-pyridin-3-ylpyrimidine |
InChI |
InChI=1S/C19H13N5/c1-3-10-21-15(7-1)18-12-17(14-6-5-9-20-13-14)23-19(24-18)16-8-2-4-11-22-16/h1-13H |
InChI Key |
NAIGHQGBSCRDHB-UHFFFAOYSA-N |
Canonical SMILES |
C1=CC=NC(=C1)C2=NC(=NC(=C2)C3=CN=CC=C3)C4=CC=CC=N4 |
Origin of Product |
United States |
Disclaimer and Information on In-Vitro Research Products
Please be aware that all articles and product information presented on BenchChem are intended solely for informational purposes. The products available for purchase on BenchChem are specifically designed for in-vitro studies, which are conducted outside of living organisms. In-vitro studies, derived from the Latin term "in glass," involve experiments performed in controlled laboratory settings using cells or tissues. It is important to note that these products are not categorized as medicines or drugs, and they have not received approval from the FDA for the prevention, treatment, or cure of any medical condition, ailment, or disease. We must emphasize that any form of bodily introduction of these products into humans or animals is strictly prohibited by law. It is essential to adhere to these guidelines to ensure compliance with legal and ethical standards in research and experimentation.