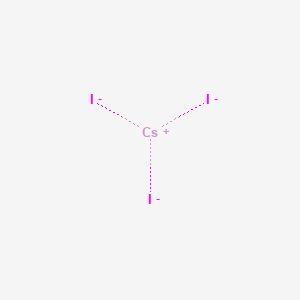
cesium;triiodide
- Click on QUICK INQUIRY to receive a quote from our team of experts.
- With the quality product at a COMPETITIVE price, you can focus more on your research.
Overview
Description
Cesium triiodide is an inorganic compound with the chemical formula CsI₃. It is known for its distinctive purple appearance and is composed of one cesium ion (Cs⁺) and three iodide ions (I⁻). This compound is notable for its unique structural and chemical properties, which make it of interest in various scientific and industrial applications.
Preparation Methods
Cesium triiodide can be synthesized through the slow volatilization and crystallization of cesium iodide and iodine in an aqueous ethanol solution . This method involves dissolving cesium iodide and iodine in ethanol, followed by the gradual evaporation of the solvent to allow the compound to crystallize. Industrial production methods may involve similar processes but on a larger scale, ensuring the purity and consistency of the final product.
Chemical Reactions Analysis
Cesium triiodide undergoes several types of chemical reactions, including:
Oxidation and Reduction: It can participate in redox reactions where the iodide ions can be oxidized to iodine or reduced to iodide ions.
Substitution Reactions: Cesium triiodide can react with other halogens or halide compounds, leading to the substitution of iodide ions with other halide ions.
Precipitation Reactions: It can form precipitates with certain organic compounds, such as diazobenzene.
Common reagents and conditions for these reactions include aqueous solutions, organic solvents like ethanol, and various halogenating agents. The major products formed from these reactions depend on the specific reactants and conditions used.
Scientific Research Applications
Cesium triiodide has several scientific research applications, including:
Chemistry: It is used in the study of inorganic compounds and their reactions, particularly in understanding the behavior of polyhalides.
Biology and Medicine:
Industry: Cesium triiodide is used in the production of scintillation detectors, which are essential in various fields such as medical imaging, security, and environmental monitoring
Mechanism of Action
The mechanism by which cesium triiodide exerts its effects is primarily through its ability to undergo phase transitions and structural changes under different conditions. For example, it undergoes a phase transition from a layered structure to a three-dimensional structure under high pressure . These structural changes can influence its chemical reactivity and physical properties, making it useful in various applications.
Comparison with Similar Compounds
Cesium triiodide can be compared with other similar compounds, such as:
Cesium iodide (CsI): Unlike cesium triiodide, cesium iodide contains only one iodide ion and is used in different applications, such as in infrared optics and as a scintillation material.
Cesium lead triiodide (CsPbI₃): This compound is used in photovoltaic applications due to its exceptional optoelectronic properties.
Cesium dibromo-iodide (CsBrI₂): Similar to cesium triiodide, this compound contains multiple halide ions and is studied for its unique structural properties.
Cesium triiodide is unique due to its specific composition and the ability to form precipitates with certain organic compounds, as well as its phase transition behavior under high pressure.
Properties
Molecular Formula |
CsI3-2 |
---|---|
Molecular Weight |
513.6189 g/mol |
IUPAC Name |
cesium;triiodide |
InChI |
InChI=1S/Cs.3HI/h;3*1H/q+1;;;/p-3 |
InChI Key |
UBARFHYEKZLYQX-UHFFFAOYSA-K |
Canonical SMILES |
[I-].[I-].[I-].[Cs+] |
Origin of Product |
United States |
Disclaimer and Information on In-Vitro Research Products
Please be aware that all articles and product information presented on BenchChem are intended solely for informational purposes. The products available for purchase on BenchChem are specifically designed for in-vitro studies, which are conducted outside of living organisms. In-vitro studies, derived from the Latin term "in glass," involve experiments performed in controlled laboratory settings using cells or tissues. It is important to note that these products are not categorized as medicines or drugs, and they have not received approval from the FDA for the prevention, treatment, or cure of any medical condition, ailment, or disease. We must emphasize that any form of bodily introduction of these products into humans or animals is strictly prohibited by law. It is essential to adhere to these guidelines to ensure compliance with legal and ethical standards in research and experimentation.