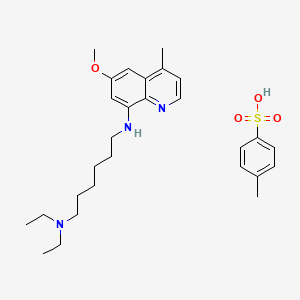
Sitamaquine tosylate
Overview
Description
Sitamaquine is an antileishmanial agent. It is active against L. donovani, L. infantum, L. mexicana, L. braziliensis, and L. tropica (EC50s = 9.5-19.8 µM). It inhibits mitochondrial complex II, also known as succinate dehydrogenase (SDH), in a cell-free assay when used at concentrations ranging from 10 to 200 µM. Sitamaquine (100 µM) increases intracellular levels of reactive oxygen species (ROS) and decreases intracellular ATP levels, as well as induces phosphatidylserine externalization, chromatin fragmentation, and depolarization of the mitochondrial membrane potential, markers of apoptosis, in L. donovani promastigotes.
Mechanism of Action
Target of Action
Sitamaquine primarily targets the Leishmania parasites . It is active against various species including L. donovani, L. infantum, L. mexicana, L. braziliensis, and L. tropica . These parasites are responsible for causing leishmaniasis, a disease that affects millions of people worldwide .
Mode of Action
Like other quinoline derivatives, it is thought to inhibitheme polymerase activity . This inhibition results in the accumulation of free heme, which is toxic to the parasites . Additionally, sitamaquine potently inhibits respiratory chain complex II , also known as succinate dehydrogenase (SDH) .
Biochemical Pathways
Sitamaquine affects the biochemical pathways related to heme metabolism and mitochondrial respiration . By inhibiting heme polymerase, it disrupts the parasite’s ability to detoxify heme, leading to its accumulation and subsequent toxicity . The inhibition of succinate dehydrogenase disrupts the normal functioning of the parasite’s respiratory chain, leading to impaired energy production .
Pharmacokinetics
It is known that sitamaquine can be taken regardless of food intake . More research is needed to fully understand the ADME (Absorption, Distribution, Metabolism, and Excretion) properties of sitamaquine .
Result of Action
Sitamaquine’s action results in the death of Leishmania parasites. It induces an apoptosis-like death in the parasites by increasing intracellular levels of reactive oxygen species (ROS), decreasing intracellular ATP levels, and inducing phosphatidylserine externalization, chromatin fragmentation, and depolarization of the mitochondrial membrane potential .
Action Environment
The action of sitamaquine is influenced by the acidic environment within the Leishmania parasites. Sitamaquine is a lipophilic weak base that rapidly accumulates in the acidic compartments of Leishmania parasites, mainly in acidocalcisomes . This allows sitamaquine to concentrate its effects where they are most needed, enhancing its efficacy .
Properties
IUPAC Name |
N',N'-diethyl-N-(6-methoxy-4-methylquinolin-8-yl)hexane-1,6-diamine;4-methylbenzenesulfonic acid | |
---|---|---|
Source | PubChem | |
URL | https://pubchem.ncbi.nlm.nih.gov | |
Description | Data deposited in or computed by PubChem | |
InChI |
InChI=1S/C21H33N3O.C7H8O3S/c1-5-24(6-2)14-10-8-7-9-12-22-20-16-18(25-4)15-19-17(3)11-13-23-21(19)20;1-6-2-4-7(5-3-6)11(8,9)10/h11,13,15-16,22H,5-10,12,14H2,1-4H3;2-5H,1H3,(H,8,9,10) | |
Source | PubChem | |
URL | https://pubchem.ncbi.nlm.nih.gov | |
Description | Data deposited in or computed by PubChem | |
InChI Key |
LOBPWLFCZDGYKW-UHFFFAOYSA-N | |
Source | PubChem | |
URL | https://pubchem.ncbi.nlm.nih.gov | |
Description | Data deposited in or computed by PubChem | |
Canonical SMILES |
CCN(CC)CCCCCCNC1=CC(=CC2=C(C=CN=C12)C)OC.CC1=CC=C(C=C1)S(=O)(=O)O | |
Source | PubChem | |
URL | https://pubchem.ncbi.nlm.nih.gov | |
Description | Data deposited in or computed by PubChem | |
Molecular Formula |
C28H41N3O4S | |
Source | PubChem | |
URL | https://pubchem.ncbi.nlm.nih.gov | |
Description | Data deposited in or computed by PubChem | |
Molecular Weight |
515.7 g/mol | |
Source | PubChem | |
URL | https://pubchem.ncbi.nlm.nih.gov | |
Description | Data deposited in or computed by PubChem | |
CAS No. |
1019640-33-5 | |
Record name | N,N-diethyl-N'-[4-methyl-6-(methyloxy)-8-quinolinyl]-1,6-hexanediamine 4-methylbenzenesulfonate | |
Source | European Chemicals Agency (ECHA) | |
URL | https://echa.europa.eu/information-on-chemicals | |
Description | The European Chemicals Agency (ECHA) is an agency of the European Union which is the driving force among regulatory authorities in implementing the EU's groundbreaking chemicals legislation for the benefit of human health and the environment as well as for innovation and competitiveness. | |
Explanation | Use of the information, documents and data from the ECHA website is subject to the terms and conditions of this Legal Notice, and subject to other binding limitations provided for under applicable law, the information, documents and data made available on the ECHA website may be reproduced, distributed and/or used, totally or in part, for non-commercial purposes provided that ECHA is acknowledged as the source: "Source: European Chemicals Agency, http://echa.europa.eu/". Such acknowledgement must be included in each copy of the material. ECHA permits and encourages organisations and individuals to create links to the ECHA website under the following cumulative conditions: Links can only be made to webpages that provide a link to the Legal Notice page. | |
Retrosynthesis Analysis
AI-Powered Synthesis Planning: Our tool employs the Template_relevance Pistachio, Template_relevance Bkms_metabolic, Template_relevance Pistachio_ringbreaker, Template_relevance Reaxys, Template_relevance Reaxys_biocatalysis model, leveraging a vast database of chemical reactions to predict feasible synthetic routes.
One-Step Synthesis Focus: Specifically designed for one-step synthesis, it provides concise and direct routes for your target compounds, streamlining the synthesis process.
Accurate Predictions: Utilizing the extensive PISTACHIO, BKMS_METABOLIC, PISTACHIO_RINGBREAKER, REAXYS, REAXYS_BIOCATALYSIS database, our tool offers high-accuracy predictions, reflecting the latest in chemical research and data.
Strategy Settings
Precursor scoring | Relevance Heuristic |
---|---|
Min. plausibility | 0.01 |
Model | Template_relevance |
Template Set | Pistachio/Bkms_metabolic/Pistachio_ringbreaker/Reaxys/Reaxys_biocatalysis |
Top-N result to add to graph | 6 |
Feasible Synthetic Routes
Disclaimer and Information on In-Vitro Research Products
Please be aware that all articles and product information presented on BenchChem are intended solely for informational purposes. The products available for purchase on BenchChem are specifically designed for in-vitro studies, which are conducted outside of living organisms. In-vitro studies, derived from the Latin term "in glass," involve experiments performed in controlled laboratory settings using cells or tissues. It is important to note that these products are not categorized as medicines or drugs, and they have not received approval from the FDA for the prevention, treatment, or cure of any medical condition, ailment, or disease. We must emphasize that any form of bodily introduction of these products into humans or animals is strictly prohibited by law. It is essential to adhere to these guidelines to ensure compliance with legal and ethical standards in research and experimentation.