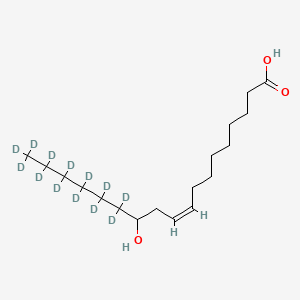
rac Ricinoleic Acid-d13
- Click on QUICK INQUIRY to receive a quote from our team of experts.
- With the quality product at a COMPETITIVE price, you can focus more on your research.
Overview
Description
Rac Ricinoleic Acid-d13 is a polyunsaturated fatty acid found primarily in castor oil. It is an isotopic analogue of rac Ricinoleic Acid, which is known for its various therapeutic properties, including anti-inflammatory, anti-oxidant, anti-bacterial, and anti-cancer effects . The molecular formula of this compound is C18H21D13O3, and it has a molecular weight of 311.54 .
Preparation Methods
Rac Ricinoleic Acid-d13 can be synthesized through the hydrolysis of castor oil, which contains approximately 90% ricinoleic acid . The hydrolysis process can be catalyzed by acids, bases, or enzymes. Enzymatic hydrolysis using lipase, such as Lipozyme TLIM, is preferred due to its efficiency and environmentally friendly nature . The optimized reaction conditions for enzymatic hydrolysis include a temperature of 41.3°C, an enzyme load of 8.9%, a hydrolysis time of 39.2 hours, and a 40:1 molar ratio of water to oil .
Chemical Reactions Analysis
Rac Ricinoleic Acid-d13 undergoes various chemical reactions, including oxidation, reduction, and substitution. Common reagents used in these reactions include oxidizing agents like potassium permanganate and reducing agents like lithium aluminum hydride. The major products formed from these reactions depend on the specific conditions and reagents used. For example, oxidation of this compound can yield products like 12-keto-9-octadecenoic acid, while reduction can produce 12-hydroxy-9-octadecenoic acid .
Scientific Research Applications
Rac Ricinoleic Acid-d13 has been utilized in various scientific research applications, including biochemistry, physiology, and pharmacology. In biochemistry, it is used to study the structure and function of proteins and enzymes. In physiology, it helps in understanding the effects of fatty acids on cell membrane function. In pharmacology, it is employed to investigate the effects of drugs on the body. Additionally, this compound has potential therapeutic applications due to its anti-inflammatory, anti-oxidant, anti-bacterial, and anti-cancer properties.
Mechanism of Action
The mechanism of action of rac Ricinoleic Acid-d13 is not fully understood. it is believed to exert its effects by inhibiting the action of certain enzymes, such as cyclooxygenase and lipoxygenase, which are involved in inflammation and oxidative stress. Additionally, this compound may modulate the expression of certain genes involved in cell proliferation and apoptosis. It also interacts with molecular targets like cannabinoid receptors and transient receptor potential cation channel subfamily V member 1 .
Comparison with Similar Compounds
Rac Ricinoleic Acid-d13 is similar to other isotopic analogues of ricinoleic acid, such as rac 12-Hydroxy-9-octadecenoic Acid-d13, rac Edenor Ri 90-d13, and rac Nouracid CS 80-d13. These compounds share similar therapeutic properties and applications. this compound is unique due to its specific isotopic labeling, which makes it particularly useful in research applications that require precise tracking and analysis of metabolic pathways.
Properties
Molecular Formula |
C18H34O3 |
---|---|
Molecular Weight |
311.5 g/mol |
IUPAC Name |
(Z)-13,13,14,14,15,15,16,16,17,17,18,18,18-tridecadeuterio-12-hydroxyoctadec-9-enoic acid |
InChI |
InChI=1S/C18H34O3/c1-2-3-4-11-14-17(19)15-12-9-7-5-6-8-10-13-16-18(20)21/h9,12,17,19H,2-8,10-11,13-16H2,1H3,(H,20,21)/b12-9-/i1D3,2D2,3D2,4D2,11D2,14D2 |
InChI Key |
WBHHMMIMDMUBKC-WZONEWBWSA-N |
Isomeric SMILES |
[2H]C([2H])([2H])C([2H])([2H])C([2H])([2H])C([2H])([2H])C([2H])([2H])C([2H])([2H])C(C/C=C\CCCCCCCC(=O)O)O |
Canonical SMILES |
CCCCCCC(CC=CCCCCCCCC(=O)O)O |
Origin of Product |
United States |
Disclaimer and Information on In-Vitro Research Products
Please be aware that all articles and product information presented on BenchChem are intended solely for informational purposes. The products available for purchase on BenchChem are specifically designed for in-vitro studies, which are conducted outside of living organisms. In-vitro studies, derived from the Latin term "in glass," involve experiments performed in controlled laboratory settings using cells or tissues. It is important to note that these products are not categorized as medicines or drugs, and they have not received approval from the FDA for the prevention, treatment, or cure of any medical condition, ailment, or disease. We must emphasize that any form of bodily introduction of these products into humans or animals is strictly prohibited by law. It is essential to adhere to these guidelines to ensure compliance with legal and ethical standards in research and experimentation.