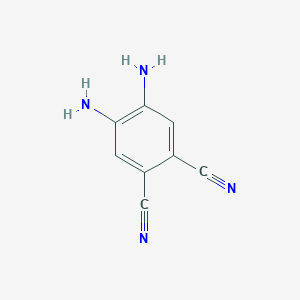
4,5-Diaminophthalonitrile
Overview
Description
4,5-Diaminophthalonitrile: is an organic compound with the molecular formula C8H6N4 1,2-diamino-4,5-dicyanobenzene or 4,5-diaminobenzene-1,2-dicarbonitrile . This compound is characterized by the presence of two amino groups and two nitrile groups attached to a benzene ring. It appears as a white to brown crystalline solid and is used as an intermediate in the synthesis of various organic compounds .
Preparation Methods
Synthetic Routes and Reaction Conditions: 4,5-Diaminophthalonitrile can be synthesized through several methods. One common method involves the reaction of 4,5-dibromo-1,2-diaminobenzene with copper cyanide . This reaction proceeds through two major steps, forming activated complexes and transition states . The reaction conditions typically involve heating the reactants in the presence of a suitable solvent and catalyst.
Industrial Production Methods: Industrial production of this compound often involves large-scale synthesis using similar methods as described above. The reaction is optimized for higher yields and purity, and the product is purified through crystallization or other separation techniques .
Chemical Reactions Analysis
Types of Reactions: 4,5-Diaminophthalonitrile undergoes various chemical reactions, including:
Oxidation: The amino groups can be oxidized to form nitro groups.
Reduction: The nitrile groups can be reduced to form amines.
Substitution: The amino groups can participate in substitution reactions with electrophiles.
Common Reagents and Conditions:
Oxidation: Reagents such as potassium permanganate or nitric acid are used under acidic or basic conditions.
Reduction: Reagents like lithium aluminum hydride or hydrogen gas in the presence of a catalyst are commonly used.
Substitution: Electrophiles such as alkyl halides or acyl chlorides are used in the presence of a base or acid catalyst.
Major Products:
Oxidation: Formation of 4,5-dinitrophthalonitrile.
Reduction: Formation of 4,5-diaminobenzene.
Substitution: Formation of various substituted derivatives depending on the electrophile used.
Scientific Research Applications
4,5-Diaminophthalonitrile has several scientific research applications, including:
Chemistry: Used as an intermediate in the synthesis of phthalocyanines, which are important dyes and pigments.
Biology: Utilized in the preparation of biologically active compounds and as a building block for drug development.
Medicine: Investigated for its potential use in the synthesis of pharmaceutical compounds with therapeutic properties.
Mechanism of Action
The mechanism of action of 4,5-diaminophthalonitrile involves its ability to participate in various chemical reactions due to the presence of amino and nitrile groups. These functional groups allow the compound to interact with different molecular targets and pathways. For example, the amino groups can form hydrogen bonds with biological molecules, while the nitrile groups can participate in nucleophilic addition reactions .
Comparison with Similar Compounds
- 4,5-Dichlorophthalonitrile
- 4,5-Diamino-2,3-pyrazinedicarbonitrile
- 4,5-Diaminobenzene-1,2-dicarbonitrile
Comparison: 4,5-Diaminophthalonitrile is unique due to the presence of both amino and nitrile groups on the benzene ring, which allows it to participate in a wide range of chemical reactions. Compared to similar compounds, it offers greater versatility in synthetic applications and can be used to produce a variety of derivatives with different properties .
Properties
IUPAC Name |
4,5-diaminobenzene-1,2-dicarbonitrile | |
---|---|---|
Source | PubChem | |
URL | https://pubchem.ncbi.nlm.nih.gov | |
Description | Data deposited in or computed by PubChem | |
InChI |
InChI=1S/C8H6N4/c9-3-5-1-7(11)8(12)2-6(5)4-10/h1-2H,11-12H2 | |
Source | PubChem | |
URL | https://pubchem.ncbi.nlm.nih.gov | |
Description | Data deposited in or computed by PubChem | |
InChI Key |
PCKAZQYWUDIFQM-UHFFFAOYSA-N | |
Source | PubChem | |
URL | https://pubchem.ncbi.nlm.nih.gov | |
Description | Data deposited in or computed by PubChem | |
Canonical SMILES |
C1=C(C(=CC(=C1N)N)C#N)C#N | |
Source | PubChem | |
URL | https://pubchem.ncbi.nlm.nih.gov | |
Description | Data deposited in or computed by PubChem | |
Molecular Formula |
C8H6N4 | |
Source | PubChem | |
URL | https://pubchem.ncbi.nlm.nih.gov | |
Description | Data deposited in or computed by PubChem | |
DSSTOX Substance ID |
DTXSID00350279 | |
Record name | 4,5-Diaminophthalonitrile | |
Source | EPA DSSTox | |
URL | https://comptox.epa.gov/dashboard/DTXSID00350279 | |
Description | DSSTox provides a high quality public chemistry resource for supporting improved predictive toxicology. | |
Molecular Weight |
158.16 g/mol | |
Source | PubChem | |
URL | https://pubchem.ncbi.nlm.nih.gov | |
Description | Data deposited in or computed by PubChem | |
CAS No. |
129365-93-1 | |
Record name | 4,5-Diaminophthalonitrile | |
Source | EPA DSSTox | |
URL | https://comptox.epa.gov/dashboard/DTXSID00350279 | |
Description | DSSTox provides a high quality public chemistry resource for supporting improved predictive toxicology. | |
Record name | 4,5-DIAMINO-1,2- BENZENEDICARBONITRILE | |
Source | European Chemicals Agency (ECHA) | |
URL | https://echa.europa.eu/information-on-chemicals | |
Description | The European Chemicals Agency (ECHA) is an agency of the European Union which is the driving force among regulatory authorities in implementing the EU's groundbreaking chemicals legislation for the benefit of human health and the environment as well as for innovation and competitiveness. | |
Explanation | Use of the information, documents and data from the ECHA website is subject to the terms and conditions of this Legal Notice, and subject to other binding limitations provided for under applicable law, the information, documents and data made available on the ECHA website may be reproduced, distributed and/or used, totally or in part, for non-commercial purposes provided that ECHA is acknowledged as the source: "Source: European Chemicals Agency, http://echa.europa.eu/". Such acknowledgement must be included in each copy of the material. ECHA permits and encourages organisations and individuals to create links to the ECHA website under the following cumulative conditions: Links can only be made to webpages that provide a link to the Legal Notice page. | |
Synthesis routes and methods
Procedure details
Retrosynthesis Analysis
AI-Powered Synthesis Planning: Our tool employs the Template_relevance Pistachio, Template_relevance Bkms_metabolic, Template_relevance Pistachio_ringbreaker, Template_relevance Reaxys, Template_relevance Reaxys_biocatalysis model, leveraging a vast database of chemical reactions to predict feasible synthetic routes.
One-Step Synthesis Focus: Specifically designed for one-step synthesis, it provides concise and direct routes for your target compounds, streamlining the synthesis process.
Accurate Predictions: Utilizing the extensive PISTACHIO, BKMS_METABOLIC, PISTACHIO_RINGBREAKER, REAXYS, REAXYS_BIOCATALYSIS database, our tool offers high-accuracy predictions, reflecting the latest in chemical research and data.
Strategy Settings
Precursor scoring | Relevance Heuristic |
---|---|
Min. plausibility | 0.01 |
Model | Template_relevance |
Template Set | Pistachio/Bkms_metabolic/Pistachio_ringbreaker/Reaxys/Reaxys_biocatalysis |
Top-N result to add to graph | 6 |
Feasible Synthetic Routes
Q1: What makes 4,5-diaminophthalonitrile particularly useful in constructing phthalocyanines and their analogues?
A1: this compound serves as a key building block for synthesizing phthalocyanines (Pcs) and related macrocycles [, , ]. Its structure, containing two amine groups adjacent to nitrile functionalities, allows it to readily participate in condensation and cyclotetramerization reactions.
Q2: How does the incorporation of this compound into polymers influence their properties?
A2: Incorporating this compound as a chain extender in polymers significantly impacts their morphology, mechanical properties, and electrical conductivity []. For example, in segmented poly(urethane urea), its inclusion alongside polycaprolactone triol and 1,3-bis(isocyanatomethyl)cyclohexane leads to the formation of crosslinked networks with enhanced tensile strength and electrical conductivity when combined with functionalized nanodiamonds [].
Q3: Beyond phthalocyanines, what other heterocyclic systems can be synthesized using this compound?
A3: this compound serves as a versatile precursor for various heterocyclic systems. It reacts with 1,3-dicarbonyl compounds to yield benzimidazole derivatives, as highlighted in []. These benzimidazoles, particularly 2-(5,6-dicyano-1H-benzo[d]imidazol-2-yl)-N-arylbenzamides, exhibit promising antifungal activities [].
Q4: Are there any studies exploring the interaction of this compound with metals?
A4: Yes, research investigates the interaction of this compound with metals, particularly in the context of cluster formation. Studies have explored sandwich clusters composed of vanadium and this compound, focusing on their structural and magnetic properties []. While specific details are limited in the provided abstract, this research avenue highlights the potential of this compound in coordination chemistry and materials science.
Q5: What analytical techniques are commonly employed to characterize compounds derived from this compound?
A5: Characterization of this compound derivatives relies on various analytical techniques. Common methods include:
- Elemental analysis: Confirms the elemental composition of synthesized compounds, verifying the success of reactions [].
- Infrared (IR) spectroscopy: Provides insights into the functional groups present in the molecules, confirming the formation of desired bonds [].
- Nuclear Magnetic Resonance (NMR) spectroscopy: Offers detailed structural information, confirming the connectivity and arrangement of atoms within the molecules [].
Disclaimer and Information on In-Vitro Research Products
Please be aware that all articles and product information presented on BenchChem are intended solely for informational purposes. The products available for purchase on BenchChem are specifically designed for in-vitro studies, which are conducted outside of living organisms. In-vitro studies, derived from the Latin term "in glass," involve experiments performed in controlled laboratory settings using cells or tissues. It is important to note that these products are not categorized as medicines or drugs, and they have not received approval from the FDA for the prevention, treatment, or cure of any medical condition, ailment, or disease. We must emphasize that any form of bodily introduction of these products into humans or animals is strictly prohibited by law. It is essential to adhere to these guidelines to ensure compliance with legal and ethical standards in research and experimentation.