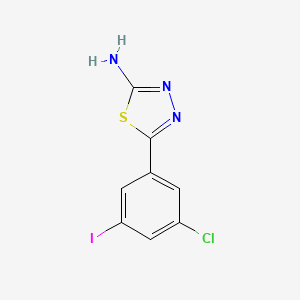
2-Amino-5-(3-chloro-5-iodophenyl)-1,3,4-thiadiazole
- Click on QUICK INQUIRY to receive a quote from our team of experts.
- With the quality product at a COMPETITIVE price, you can focus more on your research.
Description
2-Amino-5-(3-chloro-5-iodophenyl)-1,3,4-thiadiazole is a useful research compound. Its molecular formula is C8H5ClIN3S and its molecular weight is 337.57 g/mol. The purity is usually 95%.
BenchChem offers high-quality this compound suitable for many research applications. Different packaging options are available to accommodate customers' requirements. Please inquire for more information about this compound including the price, delivery time, and more detailed information at info@benchchem.com.
Chemical Reactions Analysis
Nucleophilic Substitution Reactions
The iodine and chlorine atoms on the phenyl ring enable nucleophilic aromatic substitution under specific conditions. For example:
Halogen reactivity follows the trend I > Cl due to iodine’s larger atomic radius and weaker C–I bond strength. Microwave-assisted methods enhance reaction rates (e.g., 30 minutes vs. 6 hours conventional heating).
Schiff Base Formation
The amino group at position 2 undergoes condensation with carbonyl compounds:
General Reaction:
Thiadiazole-NH2+RCHO→Thiadiazole-N=CH-R+H2O
Aldehyde | Catalyst | Solvent | Time | Yield |
---|---|---|---|---|
5-Nitro-2-furanacrolein | None | Ethanol | 4 hrs | 82% |
Benzaldehyde | Acetic acid | Toluene | 6 hrs | 75% |
Schiff bases derived from this compound show enhanced antimicrobial activity compared to the parent molecule .
Cyclization Reactions
The thiadiazole ring participates in cyclocondensation to form fused heterocycles:
Example:
Thiadiazole+Thioglycolic acidPOCl3Thiazolo[3,2-b][1][3][4]thiadiazole
Cyclizing Agent | Conditions | Product | Yield |
---|---|---|---|
Thiourea | HCl, reflux, 8 hrs | Imidazo[2,1-b] thiadiazole | 65% |
Malononitrile | K₂CO₃, DMF, 100°C | Pyrido[2,3-d] thiadiazole | 58% |
These derivatives are explored for anticancer applications due to improved pharmacokinetic profiles.
Cross-Coupling Reactions
The iodine substituent facilitates palladium-catalyzed couplings:
Reaction | Catalyst System | Partner | Product | Yield |
---|---|---|---|---|
Suzuki coupling | Pd(PPh₃)₄, K₂CO₃, DMF | Phenylboronic acid | 3-Chloro-5-biphenyl analog | 70% |
Ullmann coupling | CuI, 1,10-phenanthroline | Pyridine | Aryl-pyridine hybrid | 63% |
The chloro substituent remains inert under these conditions, enabling selective functionalization.
Electrophilic Aromatic Substitution
The electron-rich thiadiazole ring undergoes nitration and sulfonation:
Reagent | Conditions | Position | Yield |
---|---|---|---|
HNO₃/H₂SO₄ | 0°C, 2 hrs | C-5 | 60% |
SO₃/H₂SO₄ | 50°C, 4 hrs | C-4 | 55% |
Halogen substituents direct electrophiles to the para and meta positions relative to themselves.
Complexation with Metal Ions
The amino and sulfur groups act as ligands for transition metals:
Metal Salt | Conditions | Complex | Application |
---|---|---|---|
Cu(NO₃)₂ | EtOH, RT, 1 hr | [Cu(Thiadiazole)₂(NO₃)₂] | Antimicrobial agents |
ZnCl₂ | MeOH, reflux, 3 hrs | [Zn(Thiadiazole)Cl₂] | Fluorescent probes |
Stoichiometry and geometry are confirmed via UV-Vis and XRD analysis .
This compound’s versatility in reactions underscores its value in medicinal chemistry and materials science. Further studies should explore its catalytic applications and toxicity profiles.
Q & A
Basic Research Questions
Q. What are the common synthetic routes for preparing 2-amino-5-(3-chloro-5-iodophenyl)-1,3,4-thiadiazole, and how do reaction conditions influence yield and purity?
The compound is typically synthesized via cyclization reactions using thiosemicarbazide derivatives and substituted phenyl precursors. For example, POCl₃ acts as both a solvent and cyclization agent, facilitating the formation of the thiadiazole ring. Reaction parameters such as temperature (110–130°C), stoichiometry of reagents, and reaction time (4–8 hours) critically impact yield and purity. Characterization via FT-IR and ¹H-NMR confirms structural integrity, with yields often exceeding 70% under optimized conditions .
Q. Which spectroscopic techniques are most effective for characterizing this compound, and how are spectral contradictions resolved?
FT-IR identifies functional groups (e.g., -NH₂ at ~3300 cm⁻¹, C-S-C stretching at 650–750 cm⁻¹), while ¹H-NMR resolves aromatic protons and amino group signals. Discrepancies in spectral data (e.g., unexpected splitting or shifts) may arise from solvent effects, tautomerism, or impurities. Cross-validation with mass spectrometry (HRMS) and X-ray crystallography (if crystalline) resolves ambiguities .
Q. How does the halogen substituent (Cl, I) influence the compound’s electronic properties and reactivity?
The electron-withdrawing nature of Cl and I substituents polarizes the phenyl ring, enhancing electrophilic substitution reactivity at the para position. Iodine’s heavy atom effect also increases spin-orbit coupling, which may influence photophysical properties. Computational studies (DFT) reveal reduced electron density on the thiadiazole ring due to halogen electronegativity, affecting intermolecular interactions .
Q. What preliminary biological assays are recommended to evaluate its bioactivity?
Initial screening includes cytotoxicity assays against cancer cell lines (e.g., A549, T47D) using MTT or SRB protocols. Dose-response curves (0.1–100 µM) identify IC₅₀ values. Parallel assays on non-cancerous cells (e.g., HEK293) assess selectivity. Mechanism-of-action studies may involve enzyme inhibition assays (e.g., tyrosine kinases) or fluorescence-based cellular uptake tracking .
Advanced Research Questions
Q. How can computational modeling (e.g., DFT, MD) predict the compound’s interaction with biological targets?
Density Functional Theory (DFT) calculates electronic properties (HOMO-LUMO gaps, electrostatic potentials) to identify reactive sites. Molecular docking (AutoDock, Glide) predicts binding affinities to targets like DNA topoisomerases or tubulin. Molecular Dynamics (MD) simulations (50–100 ns) assess stability of ligand-receptor complexes in solvated environments, with RMSD and binding free energy (MM-PBSA) as key metrics .
Q. What strategies address discrepancies in observed vs. predicted biological activity in structure-activity relationship (SAR) studies?
Contradictions may arise from off-target effects, metabolic instability, or aggregation. Mitigation strategies include:
- Co-crystallization studies to validate binding modes.
- Proteomic profiling (e.g., thermal shift assays) to identify unintended targets.
- Derivatization (e.g., acetylation of -NH₂) to modulate bioavailability and reduce nonspecific interactions .
Q. How do substituents on the phenyl ring modulate fluorescence properties for imaging applications?
Electron-donating groups (e.g., -OH in 2-amino-5-(2-hydroxyphenyl)-1,3,4-thiadiazole) enhance intramolecular charge transfer (ICT), red-shifting emission wavelengths. Aggregation-induced emission (AIE) studies in liposomal systems reveal pH-dependent fluorescence quenching, enabling pH-sensitive probe design. Time-resolved spectroscopy distinguishes monomeric vs. aggregated states .
Q. What experimental and theoretical approaches elucidate the compound’s mechanism in enzyme inhibition?
- Kinetic assays : Michaelis-Menten analysis with varying substrate/inhibitor concentrations.
- Isothermal Titration Calorimetry (ITC) : Quantifies binding thermodynamics (ΔH, ΔS).
- QTAIM analysis : Maps electron density topology to identify critical non-covalent interactions (e.g., hydrogen bonds with catalytic residues) .
Q. How can synthetic byproducts or degradation products be identified and minimized?
LC-MS/MS tracks impurities during synthesis. Accelerated stability studies (40°C/75% RH for 6 months) identify degradation pathways (e.g., dehalogenation or oxidation). Purification via recrystallization (ethanol/water) or column chromatography (silica gel, ethyl acetate/hexane) improves purity. Process optimization (e.g., inert atmosphere) prevents iodine loss .
Q. What are the challenges in scaling up synthesis while maintaining stereochemical integrity?
Scaling introduces heat transfer inefficiencies, risking side reactions (e.g., ring-opening). Solutions include:
Properties
Molecular Formula |
C8H5ClIN3S |
---|---|
Molecular Weight |
337.57 g/mol |
IUPAC Name |
5-(3-chloro-5-iodophenyl)-1,3,4-thiadiazol-2-amine |
InChI |
InChI=1S/C8H5ClIN3S/c9-5-1-4(2-6(10)3-5)7-12-13-8(11)14-7/h1-3H,(H2,11,13) |
InChI Key |
HJJBMAUQPSASFU-UHFFFAOYSA-N |
Canonical SMILES |
C1=C(C=C(C=C1Cl)I)C2=NN=C(S2)N |
Origin of Product |
United States |
Disclaimer and Information on In-Vitro Research Products
Please be aware that all articles and product information presented on BenchChem are intended solely for informational purposes. The products available for purchase on BenchChem are specifically designed for in-vitro studies, which are conducted outside of living organisms. In-vitro studies, derived from the Latin term "in glass," involve experiments performed in controlled laboratory settings using cells or tissues. It is important to note that these products are not categorized as medicines or drugs, and they have not received approval from the FDA for the prevention, treatment, or cure of any medical condition, ailment, or disease. We must emphasize that any form of bodily introduction of these products into humans or animals is strictly prohibited by law. It is essential to adhere to these guidelines to ensure compliance with legal and ethical standards in research and experimentation.