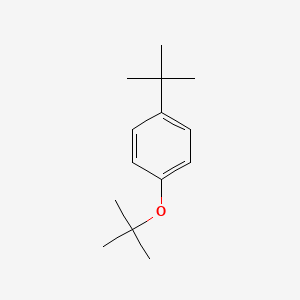
1-(tert-Butoxy)-4-(tert-butyl)benzene
- Click on QUICK INQUIRY to receive a quote from our team of experts.
- With the quality product at a COMPETITIVE price, you can focus more on your research.
Overview
Description
1-(tert-Butoxy)-4-(tert-butyl)benzene is an organic compound characterized by the presence of tert-butyl and tert-butoxy groups attached to a benzene ring
Preparation Methods
Synthetic Routes and Reaction Conditions
The synthesis of 1-(tert-Butoxy)-4-(tert-butyl)benzene typically involves the alkylation of a benzene derivative with tert-butyl and tert-butoxy groups. One common method is the Friedel-Crafts alkylation, where tert-butyl chloride and tert-butyl alcohol are used as alkylating agents in the presence of a Lewis acid catalyst such as aluminum chloride.
Industrial Production Methods
Industrial production of this compound may involve similar alkylation processes but on a larger scale. The reaction conditions are optimized to ensure high yield and purity of the product. Continuous flow reactors and advanced separation techniques are often employed to enhance efficiency and reduce production costs.
Chemical Reactions Analysis
Types of Reactions
1-(tert-Butoxy)-4-(tert-butyl)benzene undergoes various chemical reactions, including:
Oxidation: The compound can be oxidized to form corresponding quinones or other oxygenated derivatives.
Reduction: Reduction reactions can lead to the formation of hydrogenated products.
Substitution: Electrophilic aromatic substitution reactions can introduce different functional groups onto the benzene ring.
Common Reagents and Conditions
Oxidation: Common oxidizing agents include potassium permanganate and chromium trioxide.
Reduction: Hydrogen gas in the presence of a palladium catalyst is often used for reduction reactions.
Substitution: Halogenation reactions may use reagents like bromine or chlorine in the presence of a catalyst.
Major Products Formed
The major products formed from these reactions depend on the specific reagents and conditions used. For example, oxidation may yield quinones, while substitution reactions can produce halogenated derivatives.
Scientific Research Applications
1-(tert-Butoxy)-4-(tert-butyl)benzene has several applications in scientific research:
Chemistry: It is used as a precursor in the synthesis of more complex organic molecules.
Biology: The compound’s derivatives may be studied for their biological activity and potential therapeutic applications.
Medicine: Research into its pharmacological properties could lead to the development of new drugs.
Industry: It is used in the production of specialty chemicals and materials with specific properties.
Mechanism of Action
The mechanism of action of 1-(tert-Butoxy)-4-(tert-butyl)benzene involves its interaction with molecular targets through its functional groups. The tert-butyl and tert-butoxy groups can influence the compound’s reactivity and binding affinity to various substrates. Pathways involved in its action may include electrophilic and nucleophilic interactions, depending on the specific application.
Comparison with Similar Compounds
Similar Compounds
1,3,5-Tri-tert-butylbenzene: Similar in structure but with three tert-butyl groups.
tert-Butylbenzene: Contains only one tert-butyl group attached to the benzene ring.
1-tert-Butoxy-2,4,6-trimethylbenzene: Features tert-butoxy and methyl groups on the benzene ring.
Uniqueness
1-(tert-Butoxy)-4-(tert-butyl)benzene is unique due to the presence of both tert-butyl and tert-butoxy groups, which confer distinct chemical properties and reactivity patterns. This combination of functional groups makes it a valuable compound for various synthetic and industrial applications.
Properties
Molecular Formula |
C14H22O |
---|---|
Molecular Weight |
206.32 g/mol |
IUPAC Name |
1-tert-butyl-4-[(2-methylpropan-2-yl)oxy]benzene |
InChI |
InChI=1S/C14H22O/c1-13(2,3)11-7-9-12(10-8-11)15-14(4,5)6/h7-10H,1-6H3 |
InChI Key |
OQKCBQVVDYHAGJ-UHFFFAOYSA-N |
Canonical SMILES |
CC(C)(C)C1=CC=C(C=C1)OC(C)(C)C |
Origin of Product |
United States |
Disclaimer and Information on In-Vitro Research Products
Please be aware that all articles and product information presented on BenchChem are intended solely for informational purposes. The products available for purchase on BenchChem are specifically designed for in-vitro studies, which are conducted outside of living organisms. In-vitro studies, derived from the Latin term "in glass," involve experiments performed in controlled laboratory settings using cells or tissues. It is important to note that these products are not categorized as medicines or drugs, and they have not received approval from the FDA for the prevention, treatment, or cure of any medical condition, ailment, or disease. We must emphasize that any form of bodily introduction of these products into humans or animals is strictly prohibited by law. It is essential to adhere to these guidelines to ensure compliance with legal and ethical standards in research and experimentation.