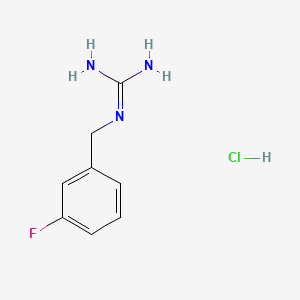
1-(3-Fluorobenzyl)guanidine Hydrochloride
- Click on QUICK INQUIRY to receive a quote from our team of experts.
- With the quality product at a COMPETITIVE price, you can focus more on your research.
Overview
Description
1-(3-Fluorobenzyl)guanidine Hydrochloride is a chemical compound with the molecular formula C8H11ClFN3 and a molecular weight of 203.65 g/mol . It is a derivative of guanidine, a strong organic base, and features a fluorobenzyl group attached to the guanidine moiety. This compound is primarily used in research and development settings.
Preparation Methods
Synthetic Routes and Reaction Conditions: The synthesis of 1-(3-Fluorobenzyl)guanidine Hydrochloride typically involves the reaction of 3-fluorobenzylamine with a guanidylating agent. One common method is the reaction of 3-fluorobenzylamine with S-methylisothiourea under basic conditions to yield the desired guanidine derivative . The reaction is usually carried out in an organic solvent such as ethanol or methanol at elevated temperatures.
Industrial Production Methods: Industrial production of guanidine derivatives, including this compound, often employs catalytic guanylation reactions. These reactions involve the use of transition metal catalysts to facilitate the formation of the C-N bond between the amine and the guanidylating agent . This method is preferred for its efficiency and scalability.
Chemical Reactions Analysis
Types of Reactions: 1-(3-Fluorobenzyl)guanidine Hydrochloride can undergo various chemical reactions, including:
Oxidation: The compound can be oxidized to form corresponding N-oxide derivatives.
Reduction: Reduction reactions can convert the guanidine group to an amine.
Substitution: The fluorobenzyl group can participate in nucleophilic substitution reactions.
Common Reagents and Conditions:
Oxidation: Common oxidizing agents include hydrogen peroxide and m-chloroperbenzoic acid.
Reduction: Reducing agents such as lithium aluminum hydride or sodium borohydride are typically used.
Substitution: Nucleophiles like amines or thiols can be used under basic conditions to substitute the fluorobenzyl group.
Major Products:
Oxidation: N-oxide derivatives.
Reduction: Amines.
Substitution: Substituted benzyl derivatives.
Scientific Research Applications
1-(3-Fluorobenzyl)guanidine Hydrochloride has a wide range of applications in scientific research:
Chemistry: It is used as a building block for the synthesis of more complex guanidine derivatives and heterocycles.
Biology: The compound is studied for its potential biological activities, including enzyme inhibition and receptor binding.
Medicine: Research is ongoing to explore its potential as a therapeutic agent, particularly in the treatment of neurological disorders.
Industry: It serves as a precursor for the synthesis of specialty chemicals and materials.
Mechanism of Action
The mechanism of action of 1-(3-Fluorobenzyl)guanidine Hydrochloride involves its interaction with molecular targets such as enzymes and receptors. The guanidine group can form hydrogen bonds and ionic interactions with amino acid residues in the active sites of enzymes, leading to inhibition or modulation of enzyme activity . Additionally, the fluorobenzyl group can enhance the compound’s binding affinity and specificity for certain receptors.
Comparison with Similar Compounds
Guanidine Hydrochloride: A simpler guanidine derivative used as a protein denaturant and in the treatment of muscle weakness.
1-(2-Fluorobenzyl)guanidine Hydrochloride: A structural isomer with similar properties but different biological activities.
1-(4-Fluorobenzyl)guanidine Hydrochloride: Another isomer with distinct chemical and biological characteristics.
Uniqueness: 1-(3-Fluorobenzyl)guanidine Hydrochloride is unique due to the position of the fluorine atom on the benzyl group, which can influence its chemical reactivity and biological activity. The specific placement of the fluorine atom can affect the compound’s ability to interact with molecular targets, making it a valuable tool in research and development.
Properties
Molecular Formula |
C8H11ClFN3 |
---|---|
Molecular Weight |
203.64 g/mol |
IUPAC Name |
2-[(3-fluorophenyl)methyl]guanidine;hydrochloride |
InChI |
InChI=1S/C8H10FN3.ClH/c9-7-3-1-2-6(4-7)5-12-8(10)11;/h1-4H,5H2,(H4,10,11,12);1H |
InChI Key |
QPZGNXRSAHJTQS-UHFFFAOYSA-N |
Canonical SMILES |
C1=CC(=CC(=C1)F)CN=C(N)N.Cl |
Origin of Product |
United States |
Disclaimer and Information on In-Vitro Research Products
Please be aware that all articles and product information presented on BenchChem are intended solely for informational purposes. The products available for purchase on BenchChem are specifically designed for in-vitro studies, which are conducted outside of living organisms. In-vitro studies, derived from the Latin term "in glass," involve experiments performed in controlled laboratory settings using cells or tissues. It is important to note that these products are not categorized as medicines or drugs, and they have not received approval from the FDA for the prevention, treatment, or cure of any medical condition, ailment, or disease. We must emphasize that any form of bodily introduction of these products into humans or animals is strictly prohibited by law. It is essential to adhere to these guidelines to ensure compliance with legal and ethical standards in research and experimentation.