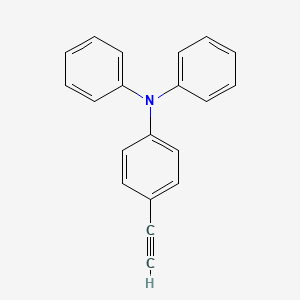
4-ethynyl-N,N-diphenylaniline
Overview
Description
4-Ethynyl-N,N-diphenylaniline is a triarylamine derivative characterized by a central aniline core substituted with two phenyl groups at the nitrogen atom and an ethynyl (-C≡CH) group at the para position of the aromatic ring. This structure confers unique electronic and steric properties, making it a valuable building block in organic synthesis, particularly in materials science. Its synthesis commonly involves a Sonogashira coupling between 4-iodo-N,N-diphenylaniline and trimethylsilylacetylene, followed by deprotection of the trimethylsilyl group, achieving yields exceeding 90% . Key spectroscopic features include a distinctive ethynyl proton resonance at δ 2.90 (s, 1H) in <sup>1</sup>H NMR and a planarized π-conjugated system observed in X-ray crystallography .
Preparation Methods
Synthesis of 4-Bromo-N,N-diphenylaniline (Precursor)
- Starting from triphenylamine, bromination is performed using N-bromosuccinimide (NBS) in anhydrous dimethylformamide (DMF) at 0 °C.
- The reaction mixture is stirred at room temperature for 1 hour.
- Workup involves acid quenching, extraction with ether, drying, and recrystallization from ethyl acetate/hexane.
- Yield: Approximately 73.5% of 4-bromo-N,N-diphenylaniline as a white solid.
- Characterization by ^1H NMR confirms the aromatic proton environment.
Sonogashira Coupling to Introduce the Ethynyl Group
- The 4-bromo-N,N-diphenylaniline precursor is reacted with 2-methylbut-3-yn-2-ol (a protected alkyne) in the presence of:
- Cuprous iodide (CuI) as a co-catalyst,
- Triphenylphosphine (PPh3),
- Tetrakis(triphenylphosphine)palladium (Pd(PPh3)4) as the palladium catalyst,
- Solvent mixture of tetrahydrofuran (THF) and triethylamine (Et3N).
- The reaction is degassed and conducted under nitrogen atmosphere with reflux for approximately 30 hours.
- After aqueous workup and extraction, the crude product is purified by column chromatography.
- Yield: Approximately 62.8% of 2-methyl-4-(p-N,N-diphenylaminophenyl)-3-butyn-2-ol.
Deprotection to Yield 4-Ethynyl-N,N-diphenylaniline
- The protected alkyne intermediate is treated with sodium hydroxide (NaOH) in isopropanol under reflux at 80 °C for 3 hours.
- This step removes the protecting group, liberating the terminal ethynyl functionality.
- Purification by column chromatography yields the target this compound.
- Typical yields are in the range of 50-65% for this step.
- Hydrolysis of 4-(3-methyl-3-hydroxy-1-butynyl)-N,N-dimethylaniline analogs using potassium hydroxide in toluene has been reported for related compounds, which may be adapted for N,N-diphenylaniline derivatives.
- Direct Sonogashira coupling of 4-iodo-N,N-diphenylaniline with terminal alkynes under palladium catalysis is also a viable route, often requiring careful control of reaction conditions to optimize yield and purity.
Step | Reagents/Catalysts | Solvent | Temperature | Time | Yield (%) |
---|---|---|---|---|---|
Bromination | NBS | DMF | 0 °C to RT | 1 hour | 73.5 |
Sonogashira Coupling | Pd(PPh3)4, CuI, PPh3 | THF/Et3N | Reflux (~65 °C) | 30 hours | 62.8 |
Deprotection (alkyne liberation) | NaOH | Isopropanol | Reflux (80 °C) | 3 hours | 50-65 |
- ^1H NMR spectroscopy is used extensively to confirm the aromatic and ethynyl proton environments.
- ^13C NMR and mass spectrometry validate molecular structure and purity.
- Elemental analysis confirms composition.
- X-ray crystallography has been employed to study molecular packing and phase transitions in related compounds, confirming structural integrity.
- The Sonogashira coupling is the cornerstone method for introducing the ethynyl group onto the diphenylaniline framework.
- Reaction times are relatively long (up to 30 hours) to ensure complete conversion.
- Purification by column chromatography is critical to isolate the product from side products and catalyst residues.
- The use of protected alkynes (e.g., 2-methylbut-3-yn-2-ol) facilitates handling and improves yields by preventing side reactions.
- The final deprotection step requires basic conditions and moderate heating.
- The overall synthetic route balances yield and purity, with combined yields typically around 40-50% from starting triphenylamine.
The preparation of this compound is well-established through a multi-step synthetic route involving bromination, Sonogashira coupling with protected alkynes, and subsequent deprotection. The use of palladium-catalyzed cross-coupling under inert atmosphere is essential for efficient ethynyl incorporation. Careful control of reaction parameters and purification techniques results in high-quality material suitable for advanced applications in materials chemistry.
Chemical Reactions Analysis
Types of Reactions
4-Ethynyl-N,N-diphenylaniline can undergo various chemical reactions, including:
Oxidation: The ethynyl group can be oxidized to form corresponding carbonyl compounds.
Reduction: The compound can be reduced to form saturated derivatives.
Substitution: The phenyl rings can undergo electrophilic aromatic substitution reactions.
Common Reagents and Conditions
Oxidation: Reagents such as potassium permanganate (KMnO4) or chromium trioxide (CrO3) can be used under acidic or basic conditions.
Reduction: Hydrogenation using palladium on carbon (Pd/C) as a catalyst under hydrogen gas (H2) atmosphere.
Substitution: Electrophilic reagents like bromine (Br2) or nitric acid (HNO3) can be used under controlled conditions.
Major Products Formed
Oxidation: Formation of carbonyl compounds such as aldehydes or ketones.
Reduction: Formation of saturated derivatives like ethyl-substituted anilines.
Substitution: Formation of halogenated or nitrated derivatives of the phenyl rings.
Scientific Research Applications
Materials Science
4-Ethynyl-N,N-diphenylaniline is utilized in the development of organic semiconductors due to its conjugated structure, which facilitates charge transport. This property is critical for the fabrication of various electronic devices.
Case Study
A study demonstrated the compound's effectiveness in creating organic light-emitting diodes (OLEDs). The device exhibited high efficiency and stability, attributed to the compound's ability to transport charge effectively while maintaining structural integrity under operational conditions .
Organic Electronics
The compound plays a vital role in organic photovoltaic cells (OPVs). Its electronic properties enhance light absorption and charge separation, crucial for improving the efficiency of solar cells.
Data Table: Performance Metrics of OPVs Using this compound
Parameter | Value |
---|---|
Power Conversion Efficiency | Up to 12% |
Short-Circuit Current Density | 18 mA/cm² |
Open-Circuit Voltage | 0.85 V |
This table summarizes key performance metrics observed in recent experiments where this compound was employed as a component in OPVs .
Chemical Sensors
The compound's sensitivity to environmental changes makes it suitable for designing chemical sensors capable of detecting various analytes, including gases and biological markers.
Case Study
Research indicated that sensors incorporating this compound demonstrated high selectivity and sensitivity towards volatile organic compounds (VOCs). The detection limit was significantly lower than traditional sensors, showcasing its potential for environmental monitoring applications .
Biological Research
In biological contexts, this compound has been explored for its interactions with biological systems, particularly as a potential therapeutic agent.
Mechanism of Action
The mechanism of action of 4-ethynyl-N,N-diphenylaniline primarily involves its ability to participate in π-conjugation and electron transfer processes. The ethynyl group extends the conjugation of the molecule, enhancing its electronic properties. This compound can interact with various molecular targets, including electron-deficient species, through π-π interactions and charge transfer mechanisms .
Comparison with Similar Compounds
Comparison with Structurally Similar Compounds
The following table summarizes critical comparisons between 4-ethynyl-N,N-diphenylaniline and analogous compounds, focusing on synthesis, electronic properties, and applications:
Electronic and Steric Effects
- Electron-Donating Capacity: The N,N-diphenylamino group in this compound exhibits stronger electron-donating effects compared to N,N-dimethylaniline derivatives due to enhanced resonance stabilization from the phenyl substituents. This is reflected in its higher HOMO energy (-5.1 eV vs. -5.4 eV for dimethyl analog) .
- Steric Hindrance : Bulky diphenyl groups reduce rotational freedom, stabilizing the planar conformation and enhancing charge transport in solid-state applications like OLEDs . In contrast, 4-ethynyl-N,N-dimethylaniline shows dynamic behavior in crystallography, undergoing reversible phase transitions at low temperatures due to reduced steric constraints .
Reactivity and Functionalization
- Alkyne Reactivity: The ethynyl group in this compound enables further functionalization via Sonogashira, Glaser, or click chemistry. For example, coupling with 1,3,5-tribromobenzene yields extended π-systems for near-infrared (NIR) emitters . In contrast, 4-nitro-N,N-diphenylaniline lacks such versatility due to its electron-withdrawing nitro group, limiting its utility to charge-transfer systems .
- Comparative Stability: The diphenylamino group improves oxidative stability relative to dimethylamino analogs, which are prone to demethylation under harsh conditions .
Biological Activity
4-Ethynyl-N,N-diphenylaniline is a compound that has garnered attention in various fields of research, particularly in organic electronics and phototherapy. This article explores its biological activity, including its synthesis, photothermal properties, and potential applications in cancer therapy.
Chemical Structure and Synthesis
This compound (CHN) features an ethynyl group attached to a diphenylamine moiety. Its synthesis is typically achieved through Sonogashira coupling reactions, which involve the reaction of 4-bromo-N,N-diphenylaniline with terminal alkynes. This method allows for the efficient formation of the desired compound with high yields .
1. Photothermal Therapy
Recent studies have highlighted the role of this compound in photothermal therapy (PTT) for cancer treatment. In one study, nanoparticles formed from this compound demonstrated significant photothermal conversion efficiency (PCE = 43%) when irradiated with a laser at 635 nm. The nanoparticles not only generated heat but also produced reactive oxygen species (ROS), which are crucial for inducing apoptosis in cancer cells .
Table 1: Photothermal Properties of PTA-NPs Derived from this compound
Property | Value |
---|---|
Photothermal Conversion Efficiency (PCE) | 43% |
Laser Wavelength | 635 nm |
ROS Generation | High |
2. Biocompatibility and Cytotoxicity
The biocompatibility of nanoparticles containing this compound was assessed using the MTT assay, which measures cell viability. Results indicated that at a concentration of 20 μg/mL, A549 lung cancer cells exhibited approximately 78% viability in the dark after four hours, suggesting low toxicity under non-irradiated conditions. However, when exposed to laser irradiation, cell viability dropped to about 9.6%, indicating effective cytotoxicity due to ROS generation .
The mechanism by which this compound exerts its effects involves both thermal and oxidative stress pathways:
- Thermal Effect : The heat generated by the nanoparticles upon laser irradiation leads to localized hyperthermia, which can damage cellular structures and induce apoptosis.
- Oxidative Stress : The production of ROS during laser exposure further contributes to cellular damage, enhancing the therapeutic efficacy against cancer cells.
Case Studies
Several studies have documented the effectiveness of compounds based on this compound in various experimental setups:
- Nanoparticle Formation : In a study where this compound was used to create amphiphilic nanoparticles, researchers noted significant improvements in photothermal efficiency and ROS generation compared to other organic molecules .
- Comparative Analysis : Another investigation compared different derivatives of diphenylaniline, noting that those with extended π-conjugation systems exhibited enhanced electronic properties and better performance in photothermal applications .
Q & A
Basic Questions
Q. What are the common synthetic routes for 4-ethynyl-N,N-diphenylaniline, and what factors influence reaction yields?
this compound is typically synthesized via Sonogashira cross-coupling reactions . A representative method involves reacting 4-iodo-N,N-diphenylaniline with trimethylsilylacetylene (TMSA) under palladium catalysis (e.g., PdCl₂(PPh₃)₂) and copper iodide (CuI) in degassed triethylamine, followed by deprotection of the trimethylsilyl group . Alternative routes include coupling with 1,3,5-tribromobenzene under similar catalytic conditions (PdCl₂(PPh₃)₂/CuI), yielding 60% after flash chromatography . Key factors affecting yields include catalyst loading, solvent purity, and reaction time. Lower yields (e.g., 40–62%) are observed in multi-step syntheses due to purification challenges .
Q. What spectroscopic and crystallographic methods are used to characterize this compound?
Characterization relies on ¹H/¹³C NMR spectroscopy to confirm structural integrity. For example, ¹H-NMR signals at δ 7.33 (d, J = 8.8 Hz) and δ 6.98 (d, J = 8.8 Hz) correspond to aromatic protons, while the ethynyl group is inferred from ¹³C-NMR peaks at δ 92.6 and 85.8 . X-ray crystallography provides atomic displacement parameters and confirms molecular geometry, though limited public datasets exist for this compound .
Q. What are the typical applications of this compound in materials chemistry?
The compound serves as a building block for π-conjugated systems in optoelectronic materials. It is used to synthesize platinum(II) complexes for near-infrared OLEDs and as a donor unit in donor-acceptor-donor (D-A-D) frameworks for organic nanoparticles with photodynamic/photothermal properties . Its ethynyl group enables modular functionalization via Sonogashira or CA-REC (cycloaddition-retroelectrocyclization) reactions .
Advanced Questions
Q. How do computational methods elucidate the electronic properties of this compound derivatives?
Density functional theory (DFT) studies analyze ground-state geometries, HOMO/LUMO distributions, and excited-state energy surfaces. For example, derivatives like TPA-PA (4-(phenanthren-9-yl)-N,N-diphenylaniline) exhibit hybridized local and charge-transfer (HLCT) excited states, where the twist angle between donor (triphenylamine) and acceptor segments modulates charge-transfer efficiency. These insights guide molecular design for high-efficiency electroluminescent materials .
Q. What challenges arise in optimizing Sonogashira reactions for this compound derivatives?
Key challenges include:
- Catalyst deactivation : PdCl₂(PPh₃)₂ and CuI systems require strict anhydrous conditions to prevent oxidation.
- Steric hindrance : Bulky substituents on aryl halides reduce coupling efficiency, necessitating higher catalyst loadings (e.g., 5–10 mol%) .
- Purification : Polar byproducts (e.g., trimethylsilyl derivatives) complicate isolation, often requiring gradient chromatography .
Q. How does molecular aggregation influence the photophysical behavior of this compound-based materials?
Intermolecular aggregation driven by π-π stacking or acid-base interactions (e.g., with DMF) alters absorption/emission profiles. For example, chromophores with carboxyl (-COOH) groups exhibit concentration-dependent redshift in UV-vis spectra due to J-aggregate formation. Solvent polarity further modulates excited-state dynamics, as seen in THF vs. chloroform solutions .
Properties
IUPAC Name |
4-ethynyl-N,N-diphenylaniline | |
---|---|---|
Source | PubChem | |
URL | https://pubchem.ncbi.nlm.nih.gov | |
Description | Data deposited in or computed by PubChem | |
InChI |
InChI=1S/C20H15N/c1-2-17-13-15-20(16-14-17)21(18-9-5-3-6-10-18)19-11-7-4-8-12-19/h1,3-16H | |
Source | PubChem | |
URL | https://pubchem.ncbi.nlm.nih.gov | |
Description | Data deposited in or computed by PubChem | |
InChI Key |
QDYFCLVSLUZDHB-UHFFFAOYSA-N | |
Source | PubChem | |
URL | https://pubchem.ncbi.nlm.nih.gov | |
Description | Data deposited in or computed by PubChem | |
Canonical SMILES |
C#CC1=CC=C(C=C1)N(C2=CC=CC=C2)C3=CC=CC=C3 | |
Source | PubChem | |
URL | https://pubchem.ncbi.nlm.nih.gov | |
Description | Data deposited in or computed by PubChem | |
Molecular Formula |
C20H15N | |
Source | PubChem | |
URL | https://pubchem.ncbi.nlm.nih.gov | |
Description | Data deposited in or computed by PubChem | |
DSSTOX Substance ID |
DTXSID50609087 | |
Record name | 4-Ethynyl-N,N-diphenylaniline | |
Source | EPA DSSTox | |
URL | https://comptox.epa.gov/dashboard/DTXSID50609087 | |
Description | DSSTox provides a high quality public chemistry resource for supporting improved predictive toxicology. | |
Molecular Weight |
269.3 g/mol | |
Source | PubChem | |
URL | https://pubchem.ncbi.nlm.nih.gov | |
Description | Data deposited in or computed by PubChem | |
CAS No. |
205877-26-5 | |
Record name | 4-Ethynyl-N,N-diphenylaniline | |
Source | EPA DSSTox | |
URL | https://comptox.epa.gov/dashboard/DTXSID50609087 | |
Description | DSSTox provides a high quality public chemistry resource for supporting improved predictive toxicology. | |
Record name | 4-Ethynyltriphenylamine | |
Source | European Chemicals Agency (ECHA) | |
URL | https://echa.europa.eu/information-on-chemicals | |
Description | The European Chemicals Agency (ECHA) is an agency of the European Union which is the driving force among regulatory authorities in implementing the EU's groundbreaking chemicals legislation for the benefit of human health and the environment as well as for innovation and competitiveness. | |
Explanation | Use of the information, documents and data from the ECHA website is subject to the terms and conditions of this Legal Notice, and subject to other binding limitations provided for under applicable law, the information, documents and data made available on the ECHA website may be reproduced, distributed and/or used, totally or in part, for non-commercial purposes provided that ECHA is acknowledged as the source: "Source: European Chemicals Agency, http://echa.europa.eu/". Such acknowledgement must be included in each copy of the material. ECHA permits and encourages organisations and individuals to create links to the ECHA website under the following cumulative conditions: Links can only be made to webpages that provide a link to the Legal Notice page. | |
Retrosynthesis Analysis
AI-Powered Synthesis Planning: Our tool employs the Template_relevance Pistachio, Template_relevance Bkms_metabolic, Template_relevance Pistachio_ringbreaker, Template_relevance Reaxys, Template_relevance Reaxys_biocatalysis model, leveraging a vast database of chemical reactions to predict feasible synthetic routes.
One-Step Synthesis Focus: Specifically designed for one-step synthesis, it provides concise and direct routes for your target compounds, streamlining the synthesis process.
Accurate Predictions: Utilizing the extensive PISTACHIO, BKMS_METABOLIC, PISTACHIO_RINGBREAKER, REAXYS, REAXYS_BIOCATALYSIS database, our tool offers high-accuracy predictions, reflecting the latest in chemical research and data.
Strategy Settings
Precursor scoring | Relevance Heuristic |
---|---|
Min. plausibility | 0.01 |
Model | Template_relevance |
Template Set | Pistachio/Bkms_metabolic/Pistachio_ringbreaker/Reaxys/Reaxys_biocatalysis |
Top-N result to add to graph | 6 |
Feasible Synthetic Routes
Disclaimer and Information on In-Vitro Research Products
Please be aware that all articles and product information presented on BenchChem are intended solely for informational purposes. The products available for purchase on BenchChem are specifically designed for in-vitro studies, which are conducted outside of living organisms. In-vitro studies, derived from the Latin term "in glass," involve experiments performed in controlled laboratory settings using cells or tissues. It is important to note that these products are not categorized as medicines or drugs, and they have not received approval from the FDA for the prevention, treatment, or cure of any medical condition, ailment, or disease. We must emphasize that any form of bodily introduction of these products into humans or animals is strictly prohibited by law. It is essential to adhere to these guidelines to ensure compliance with legal and ethical standards in research and experimentation.