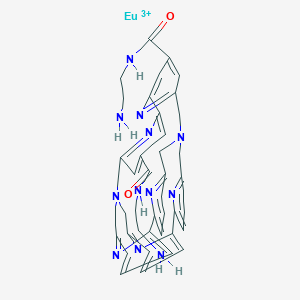
Europium(III) trisbipyridine cryptate
- Click on QUICK INQUIRY to receive a quote from our team of experts.
- With the quality product at a COMPETITIVE price, you can focus more on your research.
Overview
Description
Europium(III) trisbipyridine cryptate ([TBP(Eu³⁺)]) is a lanthanide-based complex featuring a macrocyclic "cryptand" structure with three 2,2′-bipyridine units. Developed by Nobel laureate Prof. Jean-Marie Lehn, this cryptate encapsulates the Eu³⁺ ion within a rigid, three-dimensional cage, enhancing its photophysical properties and stability . The bipyridine ligands act as "antennas," absorbing energy and transferring it to the Eu³⁺ ion, which emits long-lived fluorescence at 665 nm with a lifetime of ~300–1,000 µs . This structure minimizes non-radiative decay and environmental quenching, making it ideal for time-resolved fluorescence (TRF) applications such as:
Scientific Research Applications
Fluorescence-Based Assays
Homogeneous Time-Resolved Fluorescence (HTRF)
Europium cryptate is extensively used in HTRF technology, which enables the detection of biomolecular interactions with high precision. The long-lived emission of europium allows for time-resolved measurements that significantly reduce background noise, enhancing the sensitivity of assays.
- Applications :
Case Study: Detection of HPV DNA
A notable application involved the detection of human papillomavirus (HPV) DNA using TBP(Eu³⁺) as a fluorescent label. After PCR amplification, the hybrids were recognized by a TBP(Eu³⁺)-labeled antibody, demonstrating the compound's effectiveness in clinical diagnostics .
Biomolecule Interaction Studies
The europium cryptate's ability to serve as a donor in Förster Resonance Energy Transfer (FRET) assays has been pivotal in studying biomolecular interactions.
- Key Features :
Data Table: FRET Efficiency with TBP(Eu³⁺)
Probe Type | Emission Lifetime (ms) | FRET Efficiency (%) | Application Area |
---|---|---|---|
ODN-TBP(Eu³⁺) | 1.19 | 40 | Nucleic Acids |
Protein-TBP(Eu³⁺) | 1.15 | 35 | Immunoassays |
Labeling Reagents
This compound is also utilized as a labeling reagent for peptides, oligonucleotides, and proteins. The N-hydroxysuccinimide (NHS) activated form allows for efficient coupling to primary amines on biomolecules.
- Applications :
Enzymatic Activity Studies
The compound has been employed to study enzymatic activities through fluorescence-based methods, providing insights into enzyme kinetics and mechanisms.
Chemical Reactions Analysis
Coordination Chemistry
The europium ion in this compound exists in a +3 oxidation state, which allows it to participate in various ligand exchange reactions. In solution, europium(III) trisbipyridine cryptate can react with different anions or solvent molecules, leading to the formation of new complexes.
Ligand Exchange Reactions
The presence of bipyridine ligands facilitates strong interactions with metal ions, contributing to the stability of the complex. The ligand exchange kinetics have been studied extensively, revealing that the encapsulation of europium within cryptate cavities significantly alters its reactivity compared to free ions.
Oxidation-Reduction Reactions
Research indicates that this compound undergoes oxidation-reduction reactions, particularly involving electron transfer between Eu(III) and Eu(II) states. The encapsulated environment enhances the electron transfer rates dramatically:
-
Self-exchange reactions : The rate constants for self-exchange of europium cryptates have been found to increase significantly due to cryptate encapsulation (up to 107 times for certain complexes) compared to unencapsulated forms .
Reaction Kinetics
Kinetic studies have shown that the reactivity of europium(III) cryptates is influenced by factors such as ionic strength and the presence of complexing anions. For instance, the rate constants for various reactions involving europium(III) cryptates are summarized as follows:
Reaction Type | Rate Constant (M−1s−1) |
---|---|
Eu(2.2.1)3+/Eu(2.2.1)2+ Self-exchange | 10−4 |
Eu(2.2.2)3+/Eu(2.2.2)2+ Self-exchange | 5×10−4 |
Cross-reaction with V2+ | 10−3 |
These values indicate that encapsulation not only stabilizes the europium ion but also enhances its reactivity in outer-sphere electron transfer processes .
Immunoassays and Biomolecule Interactions
The compound has been utilized in immunoassays and biomolecule interaction studies due to its ability to provide high sensitivity and specificity:
-
TRACE Technology : This technology utilizes europium trisbipyridine cryptate for detecting molecular interactions with high temporal resolution .
-
Homogeneous Assays : The compound facilitates homogeneous assay formats that combine oligodeoxynucleotide ligation with fluorescence detection, enhancing the detection capabilities for single nucleotide polymorphisms (SNPs) .
Q & A
Basic Research Questions
Q. What are the key considerations for synthesizing Europium(III) trisbipyridine cryptate with high luminescence efficiency?
Synthesis requires precise control of bipyridine ligand substitution and cryptand encapsulation. The bipyridine units must be functionalized at the 4,4′-positions to optimize ligand-to-metal charge transfer (LMCT) and shield Eu³⁺ from solvent quenching. For example, using 4,4′-substituted bipyridine derivatives (e.g., methyl or phenyl groups) enhances photostability and quantum yield by reducing non-radiative decay pathways . Reaction conditions (pH, temperature, and Eu³⁺ concentration) must be tightly regulated to avoid incomplete cryptate formation, which can be monitored via UV-Vis spectroscopy (λmax ≈ 305 nm) .
Q. How does the bipyridine cryptand structure influence the photophysical properties of Eu³⁺ cryptate?
The trisbipyridine cryptand acts as a rigid scaffold that (i) protects Eu³⁺ from solvent interactions, (ii) enhances molar extinction coefficients (ε ≈ 30,000 M⁻¹cm⁻¹ at 305 nm), and (iii) facilitates LMCT, enabling long-lived fluorescence (τ ≈ 1 ms). The bipyridine’s conjugation length and substituents directly affect the energy gap between ligand triplet states and Eu³⁺ excited states, which governs emission intensity. For instance, electron-withdrawing groups on bipyridine lower the triplet energy, improving Förster resonance energy transfer (FRET) efficiency .
Q. What experimental setups are recommended for time-resolved fluorescence measurements using Eu³⁺ cryptate?
Use a time-resolved fluorometer with pulsed excitation (e.g., 337 nm nitrogen laser) and delayed detection (50–100 µs delay) to eliminate short-lived background fluorescence. Configure emission filters at 620 nm (direct Eu³⁺ emission) and 665 nm (FRET-acceptor emission). Calibrate using cryptate standards with known quantum yields (e.g., commercial HTRF® kits) to ensure signal linearity .
Advanced Research Questions
Q. How can energy transfer efficiency between Eu³⁺ cryptate and FRET acceptors (e.g., XL665) be optimized in heterogeneous assays?
Optimize the donor-acceptor distance (ideally 2–8 nm) and spectral overlap using Förster radius (R₀) calculations. For solid-phase assays (e.g., DNA hybridization on microtiter plates), ensure proper orientation of cryptate-labeled antibodies via maleimide-thiol conjugation to minimize steric hindrance . Validate using negative controls (e.g., unlabeled targets) to quantify non-specific energy transfer. Advanced users can employ anisotropy measurements to probe rotational freedom of the cryptate-acceptor pair .
Q. What strategies resolve contradictions in signal-to-noise ratios (SNR) when using Eu³⁺ cryptate in complex biological matrices?
- Matrix effects: Pre-treat samples with size-exclusion chromatography or protease inhibitors to remove endogenous fluorophores (e.g., hemoglobin).
- Quenching: Add chelators (e.g., EDTA) to sequester free Eu³⁺, which can cause non-specific quenching.
- Time-gated detection: Increase delay time (up to 200 µs) to suppress autofluorescence from biological components .
Validate SNR improvements using spiked recovery experiments with known analyte concentrations .
Q. How do bipyridine substituents impact cryptate stability under varying pH and ionic strength conditions?
Electron-donating substituents (e.g., -OCH₃) increase cryptate solubility in aqueous buffers but reduce stability at high ionic strength due to weakened Eu³⁺-ligand coordination. Hydrophobic groups (e.g., -CH₃) enhance stability in organic solvents but may aggregate in aqueous media. Stability can be quantified via fluorescence lifetime measurements in buffer vs. denaturing conditions (e.g., 6 M guanidine HCl) .
Q. Methodological Challenges and Data Interpretation
Q. How to troubleshoot low quantum yield in newly synthesized Eu³⁺ cryptates?
- Synthesis validation: Confirm cryptate formation via mass spectrometry and compare excitation/emission profiles to literature (e.g., λem = 620 nm).
- Solvent effects: Test in deuterated solvents to amplify LMCT efficiency via reduced vibrational quenching.
- Impurity screening: Use ICP-MS to detect free Eu³⁺, which indicates incomplete encapsulation .
Q. What computational tools aid in predicting FRET efficiency between Eu³⁺ cryptate and novel acceptors?
Use Förster theory-based software (e.g., FRETcalc or PyFRET) to model R₀ and energy transfer efficiency. Input parameters should include:
- Donor quantum yield (ΦD ≈ 0.1–0.3 for Eu³⁺ cryptate).
- Spectral overlap integral (J) from donor emission and acceptor absorption spectra.
- Orientation factor (κ² ≈ 2/3 for isotropic rotation) .
Q. Comparative and Integrative Studies
Q. How does Eu³⁺ cryptate performance compare to Terbium (Tb³⁺) cryptate in multiplexed assays?
Tb³⁺ cryptate exhibits higher quantum yield (Φ ≈ 0.6) and distinct emission peaks (λem = 490, 545, 585, 620 nm), enabling multiplexing with Eu³⁺. However, Eu³⁺ has superior Stokes shift (~300 nm vs. ~200 nm for Tb³⁺), reducing spectral crosstalk. Choose based on acceptor compatibility: Eu³⁺ pairs optimally with APC/XL665 (λem = 665 nm), while Tb³⁺ suits shorter-wavelength acceptors (e.g., D2) .
Q. Can Eu³⁺ cryptate be integrated with nanomaterials for enhanced sensing applications?
Yes. Conjugate cryptates to gold nanoparticles (AuNPs) or quantum dots (QDs) to amplify signals via plasmonic effects or secondary energy transfer. For example, AuNPs functionalized with cryptate-labeled antibodies enable ultrasensitive detection of biomarkers (LOD < 1 pM) via surface-enhanced fluorescence. Validate using TEM and dynamic light scattering to confirm nanoconjugate stability .
Comparison with Similar Compounds
Terbium(III) Cryptates
Terbium cryptates (e.g., Lumi4-Tb) share structural similarities with [TBP(Eu³⁺)] but differ in key properties:
Key Distinction : Terbium’s higher brightness and shorter-wavelength emission enable compatibility with fluorescein-like acceptors, enhancing versatility in multiplex assays .
Europium Chelates
Europium chelates (e.g., polycarboxylate complexes) lack the cryptand’s macrocyclic structure, leading to differences in stability and performance:
Key Distinction : Cryptates outperform chelates in acidic or ion-rich environments, critical for in vivo or complex biological matrices .
Other Europium(III) Complexes
- Europium Pyridine Bisbipyridine (Eu-PBP) :
- Ionic Eu³⁺ Complexes :
Lanthanide-Doped Nanoparticles
While nanoparticles (e.g., SiO₂@Eu³⁺) offer high surface area for bioconjugation, they suffer from:
- Size-dependent quenching : Reduced quantum efficiency compared to cryptates .
- Complex synthesis: Cryptates are more reproducible for clinical diagnostics .
Data Tables
Table 1: Photophysical Properties
Compound | λem (nm) | Lifetime (µs) | Quantum Yield | Reference |
---|---|---|---|---|
[TBP(Eu³⁺)] | 665 | 300–1,000 | 0.25–0.35 | |
Lumi4-Tb | 490–620 | 1,000–1,500 | 0.40–0.50 | |
Eu-PBP | 595–705 | ~200 | 0.15–0.20 | |
[Eu(terpy)₃]³⁺ | 615 | ~50 | 0.10 |
Table 2: Stability Comparison
Condition | [TBP(Eu³⁺)] | Europium Chelates |
---|---|---|
pH 3–9 | Stable | Luminescence loss |
1 mM Mg²⁺ | No ion exchange | Eu³⁺ displacement |
Serum matrix | Signal retained | Increased noise |
Properties
CAS No. |
125433-96-7 |
---|---|
Molecular Formula |
C42H42EuN12O2+3 |
Molecular Weight |
898.8 g/mol |
IUPAC Name |
5-N,10-N-bis(2-aminoethyl)-1,14,39,40,41,42,43,44-octazaoctacyclo[12.12.12.13,7.18,12.116,20.121,25.128,32.133,37]tetratetraconta-3(44),4,6,8,10,12(43),16(42),17,19,21,23,25(41),28(40),29,31,33,35,37(39)-octadecaene-5,10-dicarboxamide;europium(3+) |
InChI |
InChI=1S/C42H42N12O2.Eu/c43-13-15-45-41(55)27-17-33-25-53-21-29-5-1-9-35(47-29)36-10-2-6-30(48-36)23-54(24-32-8-4-12-38(50-32)37-11-3-7-31(22-53)49-37)26-34-18-28(42(56)46-16-14-44)20-40(52-34)39(19-27)51-33;/h1-12,17-20H,13-16,21-26,43-44H2,(H,45,55)(H,46,56);/q;+3 |
InChI Key |
GWQVMPWSEVRGPY-UHFFFAOYSA-N |
SMILES |
C1C2=NC(=CC=C2)C3=CC=CC(=N3)CN4CC5=NC(=CC=C5)C6=CC=CC(=N6)CN1CC7=NC(=CC(=C7)C(=O)NCCN)C8=CC(=CC(=N8)C4)C(=O)NCCN.[Eu+3] |
Canonical SMILES |
C1C2=NC(=CC=C2)C3=CC=CC(=N3)CN4CC5=NC(=CC=C5)C6=CC=CC(=N6)CN1CC7=NC(=CC(=C7)C(=O)NCCN)C8=CC(=CC(=N8)C4)C(=O)NCCN.[Eu+3] |
Key on ui other cas no. |
125433-96-7 |
Synonyms |
europium cryptate europium(III) cryptate europium(III) trisbipyridine cryptate |
Origin of Product |
United States |
Disclaimer and Information on In-Vitro Research Products
Please be aware that all articles and product information presented on BenchChem are intended solely for informational purposes. The products available for purchase on BenchChem are specifically designed for in-vitro studies, which are conducted outside of living organisms. In-vitro studies, derived from the Latin term "in glass," involve experiments performed in controlled laboratory settings using cells or tissues. It is important to note that these products are not categorized as medicines or drugs, and they have not received approval from the FDA for the prevention, treatment, or cure of any medical condition, ailment, or disease. We must emphasize that any form of bodily introduction of these products into humans or animals is strictly prohibited by law. It is essential to adhere to these guidelines to ensure compliance with legal and ethical standards in research and experimentation.