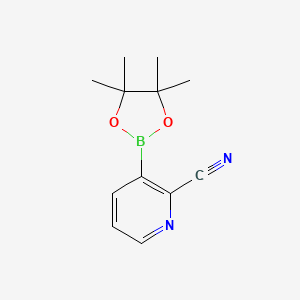
3-(4,4,5,5-Tetramethyl-1,3,2-dioxaborolan-2-yl)picolinonitrile
Overview
Description
3-(4,4,5,5-Tetramethyl-1,3,2-dioxaborolan-2-yl)picolinonitrile is a chemical compound with the molecular formula C11H16BNO2 . It appears as a white to almost white powder or crystal . It is also known as 2-(3-Pyridyl)-4,4,5,5-tetramethyl-1,3,2-dioxaborolane or 3-Pyridylboronic Acid Pinacol Ester .
Molecular Structure Analysis
The molecular structure of this compound can be analyzed using various spectroscopic techniques such as FTIR, 1H and 13C NMR spectroscopy, and mass spectrometry . The exact details of the molecular structure would require a detailed analysis of these spectroscopic data.Physical and Chemical Properties Analysis
This compound is a solid at 20°C . It is soluble in acetone and has a melting point range of 102.0 to 106.0°C .Scientific Research Applications
Synthesis and Structural Analysis
Synthesis, Crystal Structure, and Vibrational Properties Studies The compound 3-(4,4,5,5-Tetramethyl-1,3,2-dioxaborolan-2-yl)picolinonitrile has been involved in studies focused on the synthesis and structural analysis of various organic intermediates. Wu et al. (2021) synthesized and characterized the structure of related compounds using spectroscopy (FT-IR, 1H NMR, 13C NMR, and MS) and X-ray diffraction. The study also conducted Density Functional Theory (DFT) calculations to analyze spectroscopic data, geometrical parameters, molecular electrostatic potential (MEP), frontier molecular orbitals (FMO), and Natural Bond Orbital (NBO) analysis. The results showcased the consistency of molecular structures optimized by DFT with crystal structures determined by X-ray single crystal diffraction (Wu et al., 2021).
Molecular Structure and Physicochemical Properties
DFT Study of Boric Acid Ester Intermediates Another study by Huang et al. (2021) also employed a three-step substitution reaction to obtain boric acid ester intermediates with benzene rings, including the related compounds of this compound. They used FTIR, 1H and 13C NMR spectroscopy, mass spectrometry, and X-ray diffraction for structural confirmation. This study further explored the molecular electrostatic potential and frontier molecular orbitals of the compounds using DFT, revealing some of their physicochemical properties (Huang et al., 2021).
Applications in Detection and Sensing
Boronate-Based Fluorescence Probes for Hydrogen Peroxide Detection Lampard et al. (2018) synthesized a series of boronate ester fluorescence probes for detecting hydrogen peroxide (H2O2). The study highlighted the "Off–On" fluorescence response of certain probes towards H2O2, attributing this behavior to the loss of the intramolecular charge transfer (ICT) excited state. The research emphasizes the significance of incorporating electron-withdrawing or electron-donating groups in these systems for efficient sensing (Lampard et al., 2018).
Efficient Deboration Reaction for Hydrogen Peroxide Vapor Detection Fu et al. (2016) focused on enhancing the sensing performance of borate to hydrogen peroxide vapor by introducing functional groups to make the boron ester more reactive towards H2O2. Their study led to the synthesis of compounds that showed fast deboronation velocity in H2O2 vapor, significantly improving the detection limit for H2O2 vapor to as low as 4.1 parts per trillion (ppt). This research presents a valuable insight into the potential of this compound in developing sensitive detectors for hydrogen peroxide vapor (Fu et al., 2016).
Safety and Hazards
Mechanism of Action
Target of Action
Similar compounds are often used in the suzuki-miyaura cross-coupling reactions , suggesting that its targets could be various organic compounds that participate in these reactions.
Mode of Action
The compound likely interacts with its targets through a process known as borylation . In this process, the boron atom in the compound forms a bond with a carbon atom in the target molecule. This reaction is often catalyzed by a transition metal catalyst .
Biochemical Pathways
Given its potential use in suzuki-miyaura cross-coupling reactions , it may play a role in the synthesis of various organic compounds.
Result of Action
As a potential reagent in suzuki-miyaura cross-coupling reactions , it could facilitate the formation of new carbon-carbon bonds, leading to the synthesis of various organic compounds.
Action Environment
The action, efficacy, and stability of 3-(4,4,5,5-Tetramethyl-1,3,2-dioxaborolan-2-yl)picolinonitrile can be influenced by several environmental factors. For instance, the presence of a suitable catalyst is necessary for its involvement in borylation reactions . Additionally, the compound should be stored under room temperature in a cool, dry place for optimal stability .
Properties
IUPAC Name |
3-(4,4,5,5-tetramethyl-1,3,2-dioxaborolan-2-yl)pyridine-2-carbonitrile | |
---|---|---|
Source | PubChem | |
URL | https://pubchem.ncbi.nlm.nih.gov | |
Description | Data deposited in or computed by PubChem | |
InChI |
InChI=1S/C12H15BN2O2/c1-11(2)12(3,4)17-13(16-11)9-6-5-7-15-10(9)8-14/h5-7H,1-4H3 | |
Source | PubChem | |
URL | https://pubchem.ncbi.nlm.nih.gov | |
Description | Data deposited in or computed by PubChem | |
InChI Key |
CZYZGSPKMBKQBK-UHFFFAOYSA-N | |
Source | PubChem | |
URL | https://pubchem.ncbi.nlm.nih.gov | |
Description | Data deposited in or computed by PubChem | |
Canonical SMILES |
B1(OC(C(O1)(C)C)(C)C)C2=C(N=CC=C2)C#N | |
Source | PubChem | |
URL | https://pubchem.ncbi.nlm.nih.gov | |
Description | Data deposited in or computed by PubChem | |
Molecular Formula |
C12H15BN2O2 | |
Source | PubChem | |
URL | https://pubchem.ncbi.nlm.nih.gov | |
Description | Data deposited in or computed by PubChem | |
DSSTOX Substance ID |
DTXSID50580549 | |
Record name | 3-(4,4,5,5-Tetramethyl-1,3,2-dioxaborolan-2-yl)pyridine-2-carbonitrile | |
Source | EPA DSSTox | |
URL | https://comptox.epa.gov/dashboard/DTXSID50580549 | |
Description | DSSTox provides a high quality public chemistry resource for supporting improved predictive toxicology. | |
Molecular Weight |
230.07 g/mol | |
Source | PubChem | |
URL | https://pubchem.ncbi.nlm.nih.gov | |
Description | Data deposited in or computed by PubChem | |
CAS No. |
878194-93-5 | |
Record name | 3-(4,4,5,5-Tetramethyl-1,3,2-dioxaborolan-2-yl)-2-pyridinecarbonitrile | |
Source | CAS Common Chemistry | |
URL | https://commonchemistry.cas.org/detail?cas_rn=878194-93-5 | |
Description | CAS Common Chemistry is an open community resource for accessing chemical information. Nearly 500,000 chemical substances from CAS REGISTRY cover areas of community interest, including common and frequently regulated chemicals, and those relevant to high school and undergraduate chemistry classes. This chemical information, curated by our expert scientists, is provided in alignment with our mission as a division of the American Chemical Society. | |
Explanation | The data from CAS Common Chemistry is provided under a CC-BY-NC 4.0 license, unless otherwise stated. | |
Record name | 3-(4,4,5,5-Tetramethyl-1,3,2-dioxaborolan-2-yl)pyridine-2-carbonitrile | |
Source | EPA DSSTox | |
URL | https://comptox.epa.gov/dashboard/DTXSID50580549 | |
Description | DSSTox provides a high quality public chemistry resource for supporting improved predictive toxicology. | |
Record name | 3-(tetramethyl-1,3,2-dioxaborolan-2-yl)pyridine-2-carbonitrile | |
Source | European Chemicals Agency (ECHA) | |
URL | https://echa.europa.eu/information-on-chemicals | |
Description | The European Chemicals Agency (ECHA) is an agency of the European Union which is the driving force among regulatory authorities in implementing the EU's groundbreaking chemicals legislation for the benefit of human health and the environment as well as for innovation and competitiveness. | |
Explanation | Use of the information, documents and data from the ECHA website is subject to the terms and conditions of this Legal Notice, and subject to other binding limitations provided for under applicable law, the information, documents and data made available on the ECHA website may be reproduced, distributed and/or used, totally or in part, for non-commercial purposes provided that ECHA is acknowledged as the source: "Source: European Chemicals Agency, http://echa.europa.eu/". Such acknowledgement must be included in each copy of the material. ECHA permits and encourages organisations and individuals to create links to the ECHA website under the following cumulative conditions: Links can only be made to webpages that provide a link to the Legal Notice page. | |
Retrosynthesis Analysis
AI-Powered Synthesis Planning: Our tool employs the Template_relevance Pistachio, Template_relevance Bkms_metabolic, Template_relevance Pistachio_ringbreaker, Template_relevance Reaxys, Template_relevance Reaxys_biocatalysis model, leveraging a vast database of chemical reactions to predict feasible synthetic routes.
One-Step Synthesis Focus: Specifically designed for one-step synthesis, it provides concise and direct routes for your target compounds, streamlining the synthesis process.
Accurate Predictions: Utilizing the extensive PISTACHIO, BKMS_METABOLIC, PISTACHIO_RINGBREAKER, REAXYS, REAXYS_BIOCATALYSIS database, our tool offers high-accuracy predictions, reflecting the latest in chemical research and data.
Strategy Settings
Precursor scoring | Relevance Heuristic |
---|---|
Min. plausibility | 0.01 |
Model | Template_relevance |
Template Set | Pistachio/Bkms_metabolic/Pistachio_ringbreaker/Reaxys/Reaxys_biocatalysis |
Top-N result to add to graph | 6 |
Feasible Synthetic Routes
Disclaimer and Information on In-Vitro Research Products
Please be aware that all articles and product information presented on BenchChem are intended solely for informational purposes. The products available for purchase on BenchChem are specifically designed for in-vitro studies, which are conducted outside of living organisms. In-vitro studies, derived from the Latin term "in glass," involve experiments performed in controlled laboratory settings using cells or tissues. It is important to note that these products are not categorized as medicines or drugs, and they have not received approval from the FDA for the prevention, treatment, or cure of any medical condition, ailment, or disease. We must emphasize that any form of bodily introduction of these products into humans or animals is strictly prohibited by law. It is essential to adhere to these guidelines to ensure compliance with legal and ethical standards in research and experimentation.