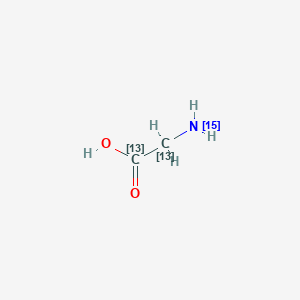
Glycine-13C2,15N
Overview
Description
Glycine-13C₂,¹⁵N is a stable isotope-labeled derivative of glycine, the simplest amino acid. It is characterized by the substitution of two carbon atoms with ¹³C and one nitrogen atom with ¹⁵N, resulting in a molecular formula of ¹³C₂H₅¹⁵NO₂ and a molecular weight of 78.05 g/mol (CAS: 211057-02-2) . This compound is synthesized to enable precise tracking of metabolic pathways, protein interactions, and biochemical reactions using techniques such as mass spectrometry (MS) and nuclear magnetic resonance (NMR) spectroscopy. Its isotopic enrichment (>95% purity) ensures minimal interference from natural abundance isotopes, making it indispensable in quantitative metabolic flux analysis and proteomics .
Preparation Methods
Synthetic Routes and Reaction Conditions
The synthesis of Glycine-13C2,15N typically involves the incorporation of isotopically labeled precursors. One common method is the reaction of isotopically labeled formaldehyde (13CH2O) with isotopically labeled ammonia (15NH3) in the presence of a catalyst to form isotopically labeled glycine . The reaction conditions often require controlled temperatures and pressures to ensure the incorporation of the isotopes into the glycine molecule.
Industrial Production Methods
Industrial production of this compound follows similar synthetic routes but on a larger scale. The process involves the use of high-purity isotopically labeled precursors and advanced catalytic systems to achieve high yields and purity of the final product. The production process is optimized to minimize the loss of isotopes and ensure the consistency of the isotopic labeling .
Chemical Reactions Analysis
Types of Reactions
Glycine-13C2,15N undergoes various chemical reactions, including:
Oxidation: this compound can be oxidized to form glyoxylate and ammonia.
Reduction: It can be reduced to form serine.
Substitution: This compound can participate in substitution reactions to form derivatives such as N-methylglycine.
Common Reagents and Conditions
Oxidation: Common oxidizing agents include hydrogen peroxide and potassium permanganate under acidic conditions.
Reduction: Reducing agents such as sodium borohydride are used under mild conditions.
Substitution: Substitution reactions often involve alkyl halides in the presence of a base.
Major Products Formed
Oxidation: Glyoxylate and ammonia.
Reduction: Serine.
Substitution: N-methylglycine and other derivatives.
Scientific Research Applications
Plant Nitrogen Uptake Studies
One of the primary applications of Glycine-13C2,15N is in studying nitrogen uptake mechanisms in plants. Research has demonstrated that plants can absorb intact glycine molecules directly from the soil, challenging the traditional view that plants rely solely on inorganic nitrogen sources such as ammonium and nitrate.
Case Study: Uptake Patterns in Tree Species
A study investigated the uptake of dual-labeled glycine (U-13C2,15N-glycine) by various tree species, including Pinus, Quercus, and Juglans. The results indicated that:
- Pinus exhibited the highest uptake rates of glycine compared to other nitrogen forms, with significant enrichment in both 13C and 15N.
- For Quercus and Larix, nitrate was the preferred nitrogen source, highlighting species-specific preferences for nitrogen forms.
- The study found that approximately 36% of glycine was absorbed intact by Pinus roots, indicating its relevance as a nitrogen source in forest ecosystems .
Soil Microbial Interactions
This compound plays a crucial role in understanding soil microbial dynamics and their interactions with plant roots. The isotopic labeling allows researchers to trace the transformation and mineralization processes of organic nitrogen sources.
Case Study: Glycine Uptake in Wheat
In field studies with wheat, researchers found that:
- At least 20% of the absorbed glycine-N was derived from intact glycine uptake.
- Gas chromatography-mass spectrometry (GC-MS) confirmed the presence of intact dual-labeled glycine in wheat roots.
- The study suggested that microbial metabolism significantly influences glycine availability and uptake efficiency by plants .
Metabolic Pathway Tracing
This compound is extensively used in metabolic studies to trace carbon and nitrogen pathways in various organisms. Its application extends to human health research, particularly in understanding amino acid metabolism and neurotransmitter synthesis.
Applications in Neurobiology
Glycine acts as an inhibitory neurotransmitter in the central nervous system (CNS). Studies utilizing labeled glycine have provided insights into:
- The synthesis pathways of neurotransmitters where glycine serves as a co-agonist alongside glutamate.
- Its role in metabolic processes related to neurodegenerative diseases .
Analytical Techniques
The use of this compound necessitates advanced analytical techniques for accurate measurement and interpretation of results. Common methods include:
- Nuclear Magnetic Resonance (NMR) : Utilized for studying molecular structures and dynamics.
- Mass Spectrometry (MS) : Essential for quantifying isotopic ratios and tracing metabolic pathways.
- Gas Chromatography-Mass Spectrometry (GC-MS) : Employed for identifying intact molecules within biological samples .
Summary Table of Applications
Application Area | Description | Key Findings |
---|---|---|
Plant Nitrogen Uptake | Investigating how plants absorb nitrogen from organic sources like glycine | Species-specific preferences for nitrogen forms; intact uptake observed in certain species |
Soil Microbial Interactions | Understanding how microbes influence nitrogen cycling and availability | Significant role of microbial metabolism in glycine uptake by plants |
Metabolic Pathway Tracing | Tracing carbon and nitrogen pathways in human health and disease research | Insights into neurotransmitter synthesis involving glycine |
Analytical Techniques | Utilizing NMR, MS, and GC-MS for precise measurement of isotopic labeling | Advanced methodologies essential for accurate data collection |
Mechanism of Action
The mechanism of action of Glycine-13C2,15N is primarily related to its role as a tracer in scientific studies. The isotopic labels allow researchers to track the movement and transformation of glycine within biological systems and chemical reactions. This enables detailed analysis of metabolic pathways, enzyme activities, and molecular interactions .
Comparison with Similar Compounds
Comparison with Similar Compounds
Glycine-13C₂,¹⁵N vs. Single-Labeled Glycine Derivatives
Glycine-¹⁵N (CAS: 20662-80-4) and Glycine-¹³C₂ (CAS: 14333-63-0) are singly labeled analogs. Glycine-13C₂,¹⁵N offers dual isotopic labeling, which enhances detection specificity in MS-based assays. For example:
- In glutathione (GSH) quantification, Glycine-13C₂,¹⁵N-labeled GSH ([¹³C₂,¹⁵N]-GSH) serves as an internal standard with distinct MRM transitions (m/z 397.2→268.2), avoiding overlap with unlabeled GSH (m/z 394.2→265.2) .
- Single-labeled derivatives (e.g., [¹⁵N]-glycine) lack the dual-signal advantage, limiting their utility in multiplexed tracer studies .
Glycine-13C₂,¹⁵N vs. Structurally Modified Isotope-Labeled Glycine Analogs
Tiglyl Glycine-13C₂,¹⁵N (CAS: 35842-45-6) is a modified glycine derivative with a tiglyl group. While it shares isotopic labels, its structural complexity (molecular weight: 160.15 g/mol) restricts its use to specialized applications, such as studying inborn errors of metabolism . In contrast, Glycine-13C₂,¹⁵N’s unmodified structure ensures compatibility with enzymatic reactions (e.g., glycine conjugation in GSH synthesis) .
Glycine-13C₂,¹⁵N vs. Non-Amino Acid Isotope-Labeled Standards
Compounds like [¹³C₈]-adenine or [¹⁵N]-guanidinoacetate are used in nucleotide or creatine metabolism studies. However, Glycine-13C₂,¹⁵N’s role as a primary amino acid allows it to integrate into broader pathways, including:
- One-carbon metabolism : Glycine contributes methyl groups via the folate cycle, tracked via ¹³C/¹⁵N enrichment .
- Antioxidant synthesis : It is directly incorporated into GSH, a critical redox regulator .
Mass Spectrometry (MS)
- Sensitivity: Glycine-13C₂,¹⁵N improves signal-to-noise ratios in LC-MS/MS by eliminating isotopic overlap. For instance, its use in GSH quantification reduces baseline interference by >90% compared to unlabeled glycine .
- Quantitative Accuracy : As an internal standard, it corrects for matrix effects in biological samples (e.g., blood, liver tissue), achieving recovery rates of 95–105% .
Nuclear Magnetic Resonance (NMR)
- Spectral Simplification : ¹³C/¹⁵N-edited ¹H-NMR decouples isotopic splitting, enabling clear detection of glycine’s α-proton (δ = 3.55 ppm) in complex mixtures .
Data Tables
Table 1: Key Properties of Glycine-13C₂,¹⁵N and Comparators
Table 2: MS Transitions for Glycine-Labeled Standards
Compound | MRM Transition (m/z) | Collision Energy (eV) |
---|---|---|
GSH-(glycine-13C₂,¹⁵N) | 397.2 → 268.2 | 20 |
Unlabeled GSH | 394.2 → 265.2 | 20 |
Research Findings
- Metabolic Tracing : In Helicobacter pylori studies, Glycine-13C₂,¹⁵N-labeled GSH revealed bacterial catabolism of host-derived GSH, with ¹³C incorporation into TCA cycle intermediates .
- Oxidative Stress Assays : Glycine-13C₂,¹⁵N enabled precise measurement of GSH/GSSG ratios in mutant yeast strains, identifying redox imbalances with ±5% error margins .
Biological Activity
Glycine-13C2,15N is a stable isotope-labeled form of glycine, an amino acid that plays a crucial role in various biological processes. This article explores its biological activity, including its metabolic pathways, physiological roles, and applications in research.
Overview of Glycine
Glycine is the simplest amino acid and serves multiple functions in human metabolism:
- Protein Synthesis : It is a building block for proteins.
- Neurotransmission : Acts as an inhibitory neurotransmitter in the central nervous system (CNS).
- Metabolic Role : Involved in the synthesis of purines and glutathione, and serves as a one-carbon donor in various metabolic reactions.
Metabolic Pathways
Glycine metabolism involves several key pathways, particularly its conversion to serine and its role in one-carbon metabolism. The use of isotopically labeled glycine allows researchers to trace these metabolic pathways effectively.
Glycine Decarboxylation and Conversion to Serine
Research has quantified the rates of glycine decarboxylation and its conversion to serine using stable isotope tracers. In a study involving healthy male volunteers, the following findings were reported:
- Glycine Decarboxylation Rate : Approximately 190 ± 41 μmol/(kg·h), accounting for about 39% of whole-body glycine flux.
- Conversion to Serine : The rate of serine synthesis from glycine was measured at 193 ± 28 μmol/(kg·h), which comprised 41% of the total glycine flux (463 ± 55 μmol/(kg·h)) .
Study on Urinary Oxalate Excretion
A study investigated the contribution of glycine metabolism to urinary oxalate excretion. Participants received primed, constant infusions of [1,2-13C2] glycine. Key results included:
- Infusion Protocol : Subjects were infused with varying doses of glycine over a period of five hours.
- Findings : The study aimed to determine whether increased plasma glycine levels would correlate with detectable levels of oxalate in urine. However, no significant conversion was detected at lower infusion rates .
Role in Plant Metabolism
This compound has also been used in agricultural studies to understand nitrogen uptake in plants. For instance:
- Experiment with Wheat : Tracer injections into soil showed how plants utilize labeled glycine for nitrogen assimilation, providing insights into nutrient cycling and plant growth .
Data Tables
The following table summarizes key findings from various studies involving this compound:
Study Focus | Methodology | Key Findings |
---|---|---|
Glycine Metabolism in Humans | Infusion studies | Decarboxylation rate: 190 μmol/(kg·h) |
Serine synthesis rate: 193 μmol/(kg·h) | ||
Urinary Oxalate Contribution | Primed constant infusion | No significant detection at low infusion rates |
Plant Nitrogen Uptake | Soil tracer injection | Demonstrated uptake preferences in wheat |
Q & A
Basic Research Questions
Q. How does the dual isotopic labeling (¹³C₂,¹⁵N) of glycine enhance precision in metabolic flux analysis?
Dual labeling allows simultaneous tracking of carbon and nitrogen atoms through metabolic pathways, enabling researchers to disentangle parallel reactions (e.g., glycine cleavage vs. serine synthesis). For example, ¹³C₂ labeling traces carbon skeleton fate in the tricarboxylic acid (TCA) cycle, while ¹⁵N tracks nitrogen redistribution into urea or purines. High-resolution liquid chromatography-mass spectrometry (HR-LC/MS) is recommended to resolve isotopic fine structure and quantify enrichment .
Q. What experimental design considerations are critical when using Glycine-¹³C₂,¹⁵N in tracer studies?
- Cell/Tissue Preparation : Use 70–80% confluent cells or 5–10 mg of tissue for reproducible extraction. Methanol/MTBE extraction preserves isotopic integrity .
- Dosing : Optimize tracer concentration to avoid isotopic dilution; for in vivo studies, intraperitoneal injection ensures systemic distribution .
- Controls : Include natural abundance glycine controls to correct for background isotopic signals .
Q. Which analytical methods are most reliable for quantifying isotopic enrichment of Glycine-¹³C₂,¹⁵N in complex biological matrices?
HR-LC/MS with a resolving power >50,000 (e.g., Orbitrap) provides accurate mass measurements to distinguish ¹³C₂,¹⁵N-glycine (m/z 76.0398) from unlabeled glycine (m/z 76.0393). Isotopic purity should be validated using gravimetrically prepared standards (e.g., 99% ¹³C and 98% ¹⁵N) .
Advanced Research Questions
Q. How can discrepancies between theoretical and observed isotopic enrichment of Glycine-¹³C₂,¹⁵N be resolved?
Discrepancies often arise from:
- Isotopic Cross-Talk : Overlapping peaks in low-resolution MS. Mitigate via chromatographic separation (e.g., HILIC columns) or mathematical correction using matrix factorization .
- Metabolic Dilution : Unlabeled glycine pools in tissues (e.g., liver) dilute the tracer. Pre-treat with isotopic equilibration phases or use compartmental modeling .
- Sample Degradation : Acidic conditions during extraction may hydrolyze glycine derivatives. Use cold methanol quenching and neutral pH buffers .
Q. What are the challenges in using Glycine-¹³C₂,¹⁵N for dynamic proteomics (e.g., SILAC) versus static metabolic labeling?
- Dynamic Labeling : Requires continuous tracer infusion to maintain isotopic equilibrium, complicating in vivo studies. Pulse-chase designs with timed sampling are advised .
- Static Labeling : Limited by cell division-dependent dilution. Use non-dividing cells (e.g., neurons) or adjust labeling duration to doubling time .
Q. How does Glycine-¹³C₂,¹⁵N improve sensitivity in detecting oxidative stress biomarkers like glutathione (GSH)?
Co-administration with ¹³C₂,¹⁵N-GSH enables isotope dilution MS for absolute quantification. For example, oxidized GSH (GSSG) can be derivatized with labeled glycine to avoid thiol-disulfide exchange artifacts. MRM transitions (e.g., m/z 394.2→265.2 for GSH; 397.2→268.2 for ¹³C₂,¹⁵N-GSH) enhance signal specificity .
Q. Methodological Insights from Recent Studies
Q. What statistical frameworks are recommended for analyzing ¹⁵N tracer partitioning in glycine metabolism?
Bayesian hierarchical models account for variability in isotopic recovery across biological replicates. For example, Markov chain Monte Carlo (MCMC) methods can estimate posterior distributions of ¹⁵N enrichment in urea versus nucleic acids, reducing Type I errors in small-sample studies .
Q. How can isotopic purity of Glycine-¹³C₂,¹⁵N impact NMR-based metabolic profiling?
Contaminants (e.g., ¹³C₁-glycine) introduce splitting in ¹³C-NMR spectra. Pre-purify via ion-exchange chromatography or use 2D NMR (HSQC) to isolate signals. Purity thresholds >98% are critical for kinetic modeling .
Q. Tables of Key Parameters
Properties
IUPAC Name |
2-(15N)azanylacetic acid | |
---|---|---|
Source | PubChem | |
URL | https://pubchem.ncbi.nlm.nih.gov | |
Description | Data deposited in or computed by PubChem | |
InChI |
InChI=1S/C2H5NO2/c3-1-2(4)5/h1,3H2,(H,4,5)/i1+1,2+1,3+1 | |
Source | PubChem | |
URL | https://pubchem.ncbi.nlm.nih.gov | |
Description | Data deposited in or computed by PubChem | |
InChI Key |
DHMQDGOQFOQNFH-VMIGTVKRSA-N | |
Source | PubChem | |
URL | https://pubchem.ncbi.nlm.nih.gov | |
Description | Data deposited in or computed by PubChem | |
Canonical SMILES |
C(C(=O)O)N | |
Source | PubChem | |
URL | https://pubchem.ncbi.nlm.nih.gov | |
Description | Data deposited in or computed by PubChem | |
Isomeric SMILES |
[13CH2]([13C](=O)O)[15NH2] | |
Source | PubChem | |
URL | https://pubchem.ncbi.nlm.nih.gov | |
Description | Data deposited in or computed by PubChem | |
Molecular Formula |
C2H5NO2 | |
Source | PubChem | |
URL | https://pubchem.ncbi.nlm.nih.gov | |
Description | Data deposited in or computed by PubChem | |
DSSTOX Substance ID |
DTXSID30583952 | |
Record name | (~13~C_2_,~15~N)Glycine | |
Source | EPA DSSTox | |
URL | https://comptox.epa.gov/dashboard/DTXSID30583952 | |
Description | DSSTox provides a high quality public chemistry resource for supporting improved predictive toxicology. | |
Molecular Weight |
78.045 g/mol | |
Source | PubChem | |
URL | https://pubchem.ncbi.nlm.nih.gov | |
Description | Data deposited in or computed by PubChem | |
CAS No. |
211057-02-2 | |
Record name | (~13~C_2_,~15~N)Glycine | |
Source | EPA DSSTox | |
URL | https://comptox.epa.gov/dashboard/DTXSID30583952 | |
Description | DSSTox provides a high quality public chemistry resource for supporting improved predictive toxicology. | |
Retrosynthesis Analysis
AI-Powered Synthesis Planning: Our tool employs the Template_relevance Pistachio, Template_relevance Bkms_metabolic, Template_relevance Pistachio_ringbreaker, Template_relevance Reaxys, Template_relevance Reaxys_biocatalysis model, leveraging a vast database of chemical reactions to predict feasible synthetic routes.
One-Step Synthesis Focus: Specifically designed for one-step synthesis, it provides concise and direct routes for your target compounds, streamlining the synthesis process.
Accurate Predictions: Utilizing the extensive PISTACHIO, BKMS_METABOLIC, PISTACHIO_RINGBREAKER, REAXYS, REAXYS_BIOCATALYSIS database, our tool offers high-accuracy predictions, reflecting the latest in chemical research and data.
Strategy Settings
Precursor scoring | Relevance Heuristic |
---|---|
Min. plausibility | 0.01 |
Model | Template_relevance |
Template Set | Pistachio/Bkms_metabolic/Pistachio_ringbreaker/Reaxys/Reaxys_biocatalysis |
Top-N result to add to graph | 6 |
Feasible Synthetic Routes
Disclaimer and Information on In-Vitro Research Products
Please be aware that all articles and product information presented on BenchChem are intended solely for informational purposes. The products available for purchase on BenchChem are specifically designed for in-vitro studies, which are conducted outside of living organisms. In-vitro studies, derived from the Latin term "in glass," involve experiments performed in controlled laboratory settings using cells or tissues. It is important to note that these products are not categorized as medicines or drugs, and they have not received approval from the FDA for the prevention, treatment, or cure of any medical condition, ailment, or disease. We must emphasize that any form of bodily introduction of these products into humans or animals is strictly prohibited by law. It is essential to adhere to these guidelines to ensure compliance with legal and ethical standards in research and experimentation.