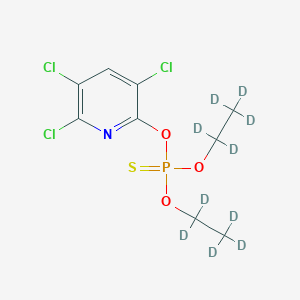
Chlorpyrifos (diethyl-D10)
Overview
Description
Chlorpyrifos: Its chemical formula is C₉D₁₀HCl₃NO₃PS with a molecular weight of 360.65 g/mol . Chlorpyrifos-d10 is commonly used as an internal standard for quantification purposes in analytical chemistry.
Scientific Research Applications
Safety and Hazards
Mechanism of Action
Target of Action
Chlorpyrifos-d10, also known as Chlorpyrifos (diethyl-D10), is an organophosphate insecticide . Its primary target is acetylcholinesterase (AChE) , a key enzyme in the nervous system of insects . AChE is responsible for the breakdown of acetylcholine, a neurotransmitter, and its inhibition leads to an accumulation of acetylcholine, causing overstimulation of the nervous system .
Mode of Action
Chlorpyrifos-d10 acts by inhibiting the activity of AChE . This inhibition disrupts the normal function of the nervous system in insects, leading to their death . The compound binds to the active site of the AChE enzyme, preventing it from breaking down acetylcholine . This results in an excess of acetylcholine in the nervous system, causing overstimulation and eventual death of the insect .
Biochemical Pathways
The biochemical pathway affected by Chlorpyrifos-d10 is the cholinergic system, specifically the pathway involving the breakdown of acetylcholine by AChE . By inhibiting AChE, Chlorpyrifos-d10 disrupts this pathway, leading to an accumulation of acetylcholine in the synapses . This causes overstimulation of the nervous system, leading to paralysis and death in insects .
Pharmacokinetics
The pharmacokinetics of Chlorpyrifos-d10 involves its absorption, distribution, metabolism, and excretion (ADME). After administration, Chlorpyrifos-d10 is absorbed and distributed throughout the body . It is metabolized primarily in the liver, where it is converted to its oxon metabolite, Chlorpyrifos-oxon . This metabolite is then excreted from the body . The pharmacokinetic properties of Chlorpyrifos-d10 can impact its bioavailability and toxicity .
Result of Action
The primary result of Chlorpyrifos-d10 action is the death of insects due to overstimulation of their nervous system . This overstimulation is caused by an accumulation of acetylcholine in the synapses, resulting from the inhibition of AChE . In addition to its insecticidal effects, Chlorpyrifos-d10 can also have impacts on non-target organisms, including potential health risks .
Action Environment
The action of Chlorpyrifos-d10 can be influenced by various environmental factors. For instance, its persistence in the environment can lead to prolonged exposure and potential bioaccumulation . Additionally, repeated applications of Chlorpyrifos-d10 can modify the soil microbial community structure . These environmental factors can influence the efficacy and stability of Chlorpyrifos-d10, as well as its impact on non-target organisms .
Biochemical Analysis
Biochemical Properties
Chlorpyrifos (diethyl-D10) plays a significant role in biochemical reactions, particularly in inhibiting acetylcholinesterase, an enzyme crucial for breaking down acetylcholine in the nervous system. The compound interacts with acetylcholinesterase by phosphorylating the serine hydroxyl group in the active site, leading to the accumulation of acetylcholine and subsequent overstimulation of cholinergic receptors . This interaction disrupts normal neurotransmission, causing neurotoxic effects.
Cellular Effects
Chlorpyrifos (diethyl-D10) affects various cell types and cellular processes. It influences cell function by disrupting cell signaling pathways, altering gene expression, and affecting cellular metabolism. The compound has been shown to induce oxidative stress, leading to the production of reactive oxygen species (ROS) and subsequent cellular damage . Additionally, Chlorpyrifos (diethyl-D10) can interfere with mitochondrial function, impairing ATP production and causing energy deficits in cells.
Molecular Mechanism
The molecular mechanism of Chlorpyrifos (diethyl-D10) involves its binding interactions with biomolecules, enzyme inhibition, and changes in gene expression. The compound inhibits acetylcholinesterase by forming a covalent bond with the enzyme’s active site, preventing the hydrolysis of acetylcholine . This inhibition leads to the accumulation of acetylcholine in synaptic clefts, resulting in continuous stimulation of cholinergic receptors and subsequent neurotoxicity. Additionally, Chlorpyrifos (diethyl-D10) can modulate the expression of genes involved in oxidative stress response and apoptosis .
Temporal Effects in Laboratory Settings
In laboratory settings, the effects of Chlorpyrifos (diethyl-D10) change over time due to its stability, degradation, and long-term impact on cellular function. The compound is relatively stable, with a half-life of approximately 385 days in soil . Over time, Chlorpyrifos (diethyl-D10) undergoes microbial degradation, leading to the formation of metabolites that may have different toxicological profiles . Long-term exposure to the compound can result in persistent oxidative stress and chronic neurotoxicity in in vitro and in vivo studies.
Dosage Effects in Animal Models
The effects of Chlorpyrifos (diethyl-D10) vary with different dosages in animal models. Low doses of the compound have been shown to increase blood glucose levels, while high doses significantly decrease blood glucose levels . Additionally, high doses of Chlorpyrifos (diethyl-D10) can cause severe neurotoxic effects, including tremors, convulsions, and even death . The compound’s toxicity is dose-dependent, with higher doses leading to more pronounced adverse effects.
Metabolic Pathways
Chlorpyrifos (diethyl-D10) is involved in several metabolic pathways, including its degradation by microbial enzymes such as organophosphorus hydrolase and methyl parathion hydrolase . These enzymes hydrolyze the P-O and P=S bonds in the compound, leading to the formation of less toxic metabolites. The metabolic pathways of Chlorpyrifos (diethyl-D10) also involve its conversion to 3,5,6-trichloro-2-pyridinol (TCP), a major metabolite that can further undergo microbial degradation .
Transport and Distribution
Chlorpyrifos (diethyl-D10) is transported and distributed within cells and tissues through various mechanisms. The compound can cross cell membranes via passive diffusion and is distributed to different tissues, including the liver, brain, and adipose tissue . Chlorpyrifos (diethyl-D10) can also bind to plasma proteins, affecting its bioavailability and distribution within the body . The compound’s lipophilic nature allows it to accumulate in fatty tissues, leading to prolonged exposure and potential toxicity.
Subcellular Localization
The subcellular localization of Chlorpyrifos (diethyl-D10) is primarily in the cytoplasm and mitochondria. The compound’s lipophilic nature allows it to penetrate cell membranes and accumulate in the mitochondrial matrix . This localization can disrupt mitochondrial function, leading to impaired ATP production and increased production of reactive oxygen species. Additionally, Chlorpyrifos (diethyl-D10) can interact with other cellular organelles, such as the endoplasmic reticulum, affecting protein synthesis and folding .
Preparation Methods
Synthetic Routes:: Chlorpyrifos-d10 can be synthesized through various routes, including the following steps:
Thiophosphorylation: Ethanol reacts with 3,5,6-trichloropyridin-2-ol to form the corresponding chloropyridinyl alcohol. This intermediate then undergoes thiophosphorylation using a phosphorodithioate reagent to yield Chlorpyrifos-d10.
Deuterium Exchange: The final step involves deuterium exchange to replace hydrogen atoms with deuterium (D) isotopes, resulting in the fully labeled compound.
Industrial Production:: Chlorpyrifos-d10 is produced on a smaller scale for research and analytical purposes. Its industrial production mainly involves custom synthesis by specialized laboratories.
Chemical Reactions Analysis
Chlorpyrifos-d10 can participate in several chemical reactions:
Hydrolysis: Under alkaline conditions, the thiophosphate group undergoes hydrolysis to yield the corresponding phosphate.
Oxidation: Chlorpyrifos-d10 can be oxidized to its oxon form, which is more toxic.
Reduction: Reduction of the chloropyridinyl moiety can occur, leading to different metabolites.
Substitution: Chlorpyrifos-d10 can undergo nucleophilic substitution reactions at the phosphorus center.
Common reagents and conditions vary depending on the specific reaction type. Major products include hydrolyzed and oxidized derivatives.
Comparison with Similar Compounds
Chlorpyrifos-d10 is unique due to its isotopic labeling, which allows precise quantification. Similar compounds include:
Chlorpyrifos: The non-labeled parent compound.
Dimethoate-d6: Another isotopically labeled pesticide used as an internal standard.
Malathion-d10: An isotopically labeled organophosphate insecticide.
Paraoxon-ethyl-d10: An isotopically labeled metabolite of Parathion.
Properties
IUPAC Name |
bis(1,1,2,2,2-pentadeuterioethoxy)-sulfanylidene-(3,5,6-trichloropyridin-2-yl)oxy-λ5-phosphane | |
---|---|---|
Source | PubChem | |
URL | https://pubchem.ncbi.nlm.nih.gov | |
Description | Data deposited in or computed by PubChem | |
InChI |
InChI=1S/C9H11Cl3NO3PS/c1-3-14-17(18,15-4-2)16-9-7(11)5-6(10)8(12)13-9/h5H,3-4H2,1-2H3/i1D3,2D3,3D2,4D2 | |
Source | PubChem | |
URL | https://pubchem.ncbi.nlm.nih.gov | |
Description | Data deposited in or computed by PubChem | |
InChI Key |
SBPBAQFWLVIOKP-MWUKXHIBSA-N | |
Source | PubChem | |
URL | https://pubchem.ncbi.nlm.nih.gov | |
Description | Data deposited in or computed by PubChem | |
Canonical SMILES |
CCOP(=S)(OCC)OC1=NC(=C(C=C1Cl)Cl)Cl | |
Source | PubChem | |
URL | https://pubchem.ncbi.nlm.nih.gov | |
Description | Data deposited in or computed by PubChem | |
Isomeric SMILES |
[2H]C([2H])([2H])C([2H])([2H])OP(=S)(OC1=NC(=C(C=C1Cl)Cl)Cl)OC([2H])([2H])C([2H])([2H])[2H] | |
Source | PubChem | |
URL | https://pubchem.ncbi.nlm.nih.gov | |
Description | Data deposited in or computed by PubChem | |
Molecular Formula |
C9H11Cl3NO3PS | |
Source | PubChem | |
URL | https://pubchem.ncbi.nlm.nih.gov | |
Description | Data deposited in or computed by PubChem | |
DSSTOX Substance ID |
DTXSID80583927 | |
Record name | O,O-Bis[(~2~H_5_)ethyl] O-(3,5,6-trichloropyridin-2-yl) phosphorothioate | |
Source | EPA DSSTox | |
URL | https://comptox.epa.gov/dashboard/DTXSID80583927 | |
Description | DSSTox provides a high quality public chemistry resource for supporting improved predictive toxicology. | |
Molecular Weight |
360.6 g/mol | |
Source | PubChem | |
URL | https://pubchem.ncbi.nlm.nih.gov | |
Description | Data deposited in or computed by PubChem | |
CAS No. |
285138-81-0 | |
Record name | O,O-Bis[(~2~H_5_)ethyl] O-(3,5,6-trichloropyridin-2-yl) phosphorothioate | |
Source | EPA DSSTox | |
URL | https://comptox.epa.gov/dashboard/DTXSID80583927 | |
Description | DSSTox provides a high quality public chemistry resource for supporting improved predictive toxicology. | |
Record name | 285138-81-0 | |
Source | European Chemicals Agency (ECHA) | |
URL | https://echa.europa.eu/information-on-chemicals | |
Description | The European Chemicals Agency (ECHA) is an agency of the European Union which is the driving force among regulatory authorities in implementing the EU's groundbreaking chemicals legislation for the benefit of human health and the environment as well as for innovation and competitiveness. | |
Explanation | Use of the information, documents and data from the ECHA website is subject to the terms and conditions of this Legal Notice, and subject to other binding limitations provided for under applicable law, the information, documents and data made available on the ECHA website may be reproduced, distributed and/or used, totally or in part, for non-commercial purposes provided that ECHA is acknowledged as the source: "Source: European Chemicals Agency, http://echa.europa.eu/". Such acknowledgement must be included in each copy of the material. ECHA permits and encourages organisations and individuals to create links to the ECHA website under the following cumulative conditions: Links can only be made to webpages that provide a link to the Legal Notice page. | |
Retrosynthesis Analysis
AI-Powered Synthesis Planning: Our tool employs the Template_relevance Pistachio, Template_relevance Bkms_metabolic, Template_relevance Pistachio_ringbreaker, Template_relevance Reaxys, Template_relevance Reaxys_biocatalysis model, leveraging a vast database of chemical reactions to predict feasible synthetic routes.
One-Step Synthesis Focus: Specifically designed for one-step synthesis, it provides concise and direct routes for your target compounds, streamlining the synthesis process.
Accurate Predictions: Utilizing the extensive PISTACHIO, BKMS_METABOLIC, PISTACHIO_RINGBREAKER, REAXYS, REAXYS_BIOCATALYSIS database, our tool offers high-accuracy predictions, reflecting the latest in chemical research and data.
Strategy Settings
Precursor scoring | Relevance Heuristic |
---|---|
Min. plausibility | 0.01 |
Model | Template_relevance |
Template Set | Pistachio/Bkms_metabolic/Pistachio_ringbreaker/Reaxys/Reaxys_biocatalysis |
Top-N result to add to graph | 6 |
Feasible Synthetic Routes
Q1: Why is Chlorpyrifos (diethyl-D10) used as an internal standard in this analytical method?
A1: The abstract states that Chlorpyrifos (diethyl-D10) is added to blood samples as an internal standard []. Internal standards are crucial in analytical chemistry, especially in gas chromatography-mass spectrometry (GC-MS) analysis. They are chemically similar to the target analytes but distinguishable by the detector. Adding a known amount of an internal standard helps account for variations during sample preparation and analysis, such as losses during extraction or injection volume fluctuations. By comparing the signal response of the target analytes to the internal standard, the method achieves higher accuracy and precision in quantifying the organophosphates in the blood samples.
Q2: What are the advantages of using this method to determine organophosphate exposure?
A2: The described method offers several advantages for biomonitoring organophosphate exposure:
- Sensitivity: The method boasts a low detection limit (0.5-2.6 µg/L) for various organophosphates, enabling the detection of even low-level occupational exposures [].
- Quantitative Analysis: The use of calibration curves generated with spiked human blood samples allows for accurate quantification of organophosphates in real-world samples [].
Disclaimer and Information on In-Vitro Research Products
Please be aware that all articles and product information presented on BenchChem are intended solely for informational purposes. The products available for purchase on BenchChem are specifically designed for in-vitro studies, which are conducted outside of living organisms. In-vitro studies, derived from the Latin term "in glass," involve experiments performed in controlled laboratory settings using cells or tissues. It is important to note that these products are not categorized as medicines or drugs, and they have not received approval from the FDA for the prevention, treatment, or cure of any medical condition, ailment, or disease. We must emphasize that any form of bodily introduction of these products into humans or animals is strictly prohibited by law. It is essential to adhere to these guidelines to ensure compliance with legal and ethical standards in research and experimentation.