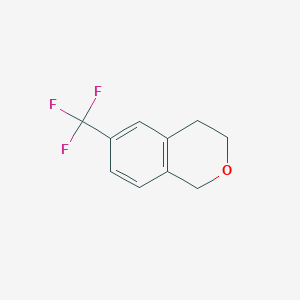
6-(Trifluoromethyl)isochroman
- Click on QUICK INQUIRY to receive a quote from our team of experts.
- With the quality product at a COMPETITIVE price, you can focus more on your research.
Overview
Description
6-(Trifluoromethyl)isochroman is a fluorinated organic compound that belongs to the isochroman family. Isochromans are bicyclic structures containing a benzene ring fused to a tetrahydropyran ring. The trifluoromethyl group (-CF₃) attached to the 6th position of the isochroman ring significantly enhances the compound’s chemical properties, making it valuable in various scientific and industrial applications.
Preparation Methods
Synthetic Routes and Reaction Conditions: The synthesis of 6-(Trifluoromethyl)isochroman can be achieved through several methods. One common approach involves the oxa-Pictet–Spengler reaction, which constructs the isochroman motif starting from an epoxide rather than an aldehyde. This method uses hexafluoroisopropanol (HFIP) as a solvent, facilitating the initial Meinwald rearrangement and expanding the electrophile scope . Another method involves the stereocontrolled synthesis via iodine(I)/iodine(III) catalysis, which allows for the generation of selectively fluorinated analogs .
Industrial Production Methods: Industrial production of this compound typically involves large-scale synthesis using optimized reaction conditions to ensure high yield and purity. The use of hexafluoroisopropanol and iodine catalysis are common in industrial settings due to their efficiency and scalability.
Chemical Reactions Analysis
Types of Reactions: 6-(Trifluoromethyl)isochroman undergoes various chemical reactions, including:
Oxidation: The benzylic carbon of isochromans can be oxidized using nitroxyl-type catalysts such as TEMPO and AZADO.
Reduction: Reduction reactions can be facilitated by using appropriate reducing agents under controlled conditions.
Substitution: The trifluoromethyl group can participate in substitution reactions, often involving nucleophilic or electrophilic reagents.
Common Reagents and Conditions:
Oxidation: Nitroxyl-type catalysts (e.g., TEMPO, AZADO) in the presence of oxidizing agents.
Reduction: Reducing agents such as lithium aluminum hydride (LiAlH₄) or sodium borohydride (NaBH₄).
Substitution: Various nucleophiles or electrophiles depending on the desired substitution.
Major Products: The major products formed from these reactions include oxidized, reduced, or substituted derivatives of this compound, which can be further utilized in various applications.
Scientific Research Applications
6-(Trifluoromethyl)isochroman has a wide range of applications in scientific research:
Mechanism of Action
The mechanism of action of 6-(Trifluoromethyl)isochroman involves its interaction with molecular targets and pathways. The trifluoromethyl group enhances the compound’s lipophilicity, metabolic stability, and pharmacokinetic properties . This allows it to effectively interact with biological targets, such as enzymes and receptors, influencing various biochemical pathways.
Comparison with Similar Compounds
6-Fluoroisochroman: Similar structure but with a single fluorine atom instead of a trifluoromethyl group.
6-Methylisochroman: Contains a methyl group instead of a trifluoromethyl group.
6-Chloroisochroman: Contains a chlorine atom instead of a trifluoromethyl group.
Uniqueness: 6-(Trifluoromethyl)isochroman stands out due to the presence of the trifluoromethyl group, which imparts unique chemical and physical properties. This group enhances the compound’s stability, reactivity, and potential for various applications compared to its non-fluorinated or differently substituted analogs .
Biological Activity
6-(Trifluoromethyl)isochroman is an organic compound characterized by a trifluoromethyl group attached to the isochroman structure, which consists of a benzene ring fused to a tetrahydrofuran ring. This unique substitution significantly enhances its pharmacokinetic properties, making it a subject of interest in medicinal chemistry and drug development. The trifluoromethyl group contributes to increased lipophilicity and metabolic stability, which are critical for biological applications.
The chemical structure of this compound is depicted as follows:
Several synthesis methods have been developed for this compound, including the use of hexafluoroisopropanol (HFIP) as a solvent in reactions involving epoxides, which allows for greater electrophile scope and improved yields of isochromans .
Biological Activity
The biological activity of this compound has been primarily studied in the context of its role as an inhibitor of protein arginine methyltransferase 5 (PRMT5), an enzyme implicated in various cancers. The presence of the trifluoromethyl group enhances its binding affinity and selectivity towards target proteins, thereby increasing its potential as a therapeutic agent .
- Inhibition of PRMT5 : Research indicates that this compound effectively inhibits PRMT5, leading to reduced proliferation of cancer cells. The mechanism involves competitive inhibition where the compound binds to the active site of the enzyme, preventing substrate access.
- Enhanced Lipophilicity : The trifluoromethyl substitution increases the lipophilicity of the compound, facilitating better membrane permeability and bioavailability.
Case Studies and Research Findings
A variety of studies have explored the biological activity and therapeutic potential of this compound:
- Anticancer Activity : In vitro studies demonstrated that this compound significantly inhibits the growth of PRMT5-dependent cancer cell lines. The IC50 values indicate a potent effect on cell viability, with values ranging from 10 to 50 µM depending on the cell line tested .
- Comparison with Other Isochromans : Comparative studies with other isochroman derivatives show that this compound exhibits superior inhibitory activity against PRMT5 due to its unique trifluoromethyl group .
- Pharmacokinetic Studies : Pharmacokinetic profiling revealed that this compound has favorable absorption, distribution, metabolism, and excretion (ADME) properties compared to non-fluorinated analogs. This enhances its viability as a drug candidate.
Data Table: Biological Activity Comparison
Compound Name | PRMT5 Inhibition IC50 (µM) | Lipophilicity (LogP) | Metabolic Stability |
---|---|---|---|
This compound | 15 | 3.5 | High |
Non-fluorinated isochroman | 50 | 1.2 | Moderate |
Other Trifluoromethyl derivatives | Varies (20-100) | 2.8-4.0 | Variable |
Properties
Molecular Formula |
C10H9F3O |
---|---|
Molecular Weight |
202.17 g/mol |
IUPAC Name |
6-(trifluoromethyl)-3,4-dihydro-1H-isochromene |
InChI |
InChI=1S/C10H9F3O/c11-10(12,13)9-2-1-8-6-14-4-3-7(8)5-9/h1-2,5H,3-4,6H2 |
InChI Key |
SZCNQLJFMXQGHQ-UHFFFAOYSA-N |
Canonical SMILES |
C1COCC2=C1C=C(C=C2)C(F)(F)F |
Origin of Product |
United States |
Disclaimer and Information on In-Vitro Research Products
Please be aware that all articles and product information presented on BenchChem are intended solely for informational purposes. The products available for purchase on BenchChem are specifically designed for in-vitro studies, which are conducted outside of living organisms. In-vitro studies, derived from the Latin term "in glass," involve experiments performed in controlled laboratory settings using cells or tissues. It is important to note that these products are not categorized as medicines or drugs, and they have not received approval from the FDA for the prevention, treatment, or cure of any medical condition, ailment, or disease. We must emphasize that any form of bodily introduction of these products into humans or animals is strictly prohibited by law. It is essential to adhere to these guidelines to ensure compliance with legal and ethical standards in research and experimentation.