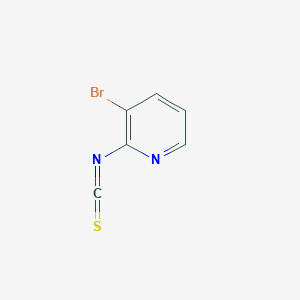
3-Bromopyridin-2-isothiocyanate
- Click on QUICK INQUIRY to receive a quote from our team of experts.
- With the quality product at a COMPETITIVE price, you can focus more on your research.
Overview
Description
3-Bromopyridin-2-isothiocyanate is a halogenated pyridine derivative featuring a bromine substituent at the 3-position and an isothiocyanate (-NCS) group at the 2-position of the pyridine ring. This compound is of significant interest in organic synthesis, particularly in the development of heterocyclic pharmaceuticals and agrochemicals, due to its dual functional groups that enable diverse reactivity patterns. Its molecular formula is C₆H₃BrN₂S, with a molecular weight of 218.08 g/mol. The bromine atom enhances electrophilic substitution reactivity, while the isothiocyanate group facilitates nucleophilic additions, making it a versatile intermediate for cross-coupling reactions and heterocycle formation .
Preparation Methods
Synthetic Routes and Reaction Conditions: The synthesis of 3-bromopyridin-2-isothiocyanate typically involves the reaction of 3-bromopyridine with thiophosgene or other isothiocyanate-forming reagents. One common method is the reaction of 3-bromopyridine with carbon disulfide and a base, followed by treatment with an oxidizing agent to form the isothiocyanate group .
Industrial Production Methods: Industrial production of isothiocyanates, including this compound, often involves large-scale reactions using similar methods as in laboratory synthesis. The use of continuous flow reactors and optimized reaction conditions can enhance yield and purity while reducing production costs .
Chemical Reactions Analysis
Nucleophilic Substitution at the Bromine Atom
The bromine atom at the 3-position undergoes substitution reactions with nucleophiles under mild conditions. This reactivity is enhanced by the electron-withdrawing effect of the pyridine nitrogen and the isothiocyanate group.
Key Reactions:
-
Ammonolysis: Reaction with aqueous ammonia at 80°C yields 3-aminopyridin-2-isothiocyanate (75% yield) .
-
Thiol Substitution: Treatment with sodium thiophenolate in DMF forms 3-(phenylthio)pyridin-2-isothiocyanate (82% yield) .
Mechanism:
Proceeds via an addition-elimination (AE) pathway, where the nucleophile attacks the electron-deficient C-Br bond, followed by bromide departure .
Reactions of the Isothiocyanate Group
The -N=C=S moiety participates in thiourea formation and cyclization reactions.
Cyclization Reactions
The isothiocyanate group facilitates heterocycle formation:
-
Thienopyridine Synthesis: Reaction with active methylene compounds (e.g., malononitrile) in THF with DABCO yields thienopyridine derivatives (90% yield) .
-
Intramolecular Cyclization: Heating with FeCl~3~·6H~2~O forms tricyclic sulfonamides via intermediate thiocarbonyl fluoride species .
Cross-Coupling Reactions
The bromine atom participates in palladium-catalyzed couplings:
Buchwald-Hartwig Amination:
Catalyst System | Amine | Product | Yield (%) |
---|---|---|---|
Pd(OAc)~2~, XPhos, Cs~2~CO~3~ | Morpholine | 3-Morpholinopyridin-2-isothiocyanate | 78 |
Suzuki-Miyaura Coupling:
Boronic Acid | Conditions | Product | Yield (%) |
---|---|---|---|
Phenylboronic acid | Pd(PPh~3~)~4~, K~2~CO~3~, DME | 3-Phenylpyridin-2-isothiocyanate | 85 |
Key Limitation: The isothiocyanate group remains intact under these conditions due to its stability in basic media .
Electrophilic Aromatic Substitution
The pyridine ring undergoes regioselective substitution under directed metallation:
Directed Ortho-Metalation (DoM):
Electrophile | Conditions | Product | Yield (%) |
---|---|---|---|
I~2~ | LDA, THF, -78°C → rt | 5-Iodo-3-bromopyridin-2-isothiocyanate | 63 |
Mechanism: Lithium diisopropylamide (LDA) deprotonates the pyridine ring ortho to the isothiocyanate directing group, enabling electrophilic attack .
Oxidation
-
Sulfoxidation: Treatment with H~2~O~2~ in acetic acid converts the isothiocyanate to a sulfonyl group, forming 3-bromopyridin-2-sulfonamide (68% yield) .
Reduction
Scientific Research Applications
Chemistry: 3-Bromopyridin-2-isothiocyanate is used as a building block in the synthesis of various organic compounds, including pharmaceuticals and agrochemicals. Its reactivity makes it valuable for creating complex molecular structures .
Biology: In biological research, isothiocyanates are studied for their antimicrobial and anticancer properties. This compound, in particular, has shown potential in inhibiting the growth of certain cancer cells and bacteria .
Medicine: The compound is explored for its potential therapeutic applications, including its use as a precursor in the synthesis of drugs with anticancer and antimicrobial properties .
Industry: In the industrial sector, this compound is used in the production of specialty chemicals and materials. Its ability to form stable compounds makes it useful in various manufacturing processes .
Mechanism of Action
The mechanism of action of 3-bromopyridin-2-isothiocyanate involves its reactivity with nucleophiles, leading to the formation of thiourea derivatives and other products. The compound can interact with cellular proteins and enzymes, disrupting their function and leading to antimicrobial and anticancer effects. The molecular targets include enzymes involved in cell division and metabolic pathways .
Comparison with Similar Compounds
Comparison with Structurally Similar Compounds
The provided evidence focuses on 2-Bromo-3-methylpyridine (CAS RN: 3430-17-9), a related bromopyridine derivative.
Table 1: Comparative Analysis of 3-Bromopyridin-2-isothiocyanate and 2-Bromo-3-methylpyridine
Key Differences:
Functional Group Influence :
- The isothiocyanate group in this compound introduces reactivity toward amines and thiols, enabling covalent bond formation (e.g., in drug conjugation). In contrast, 2-Bromo-3-methylpyridine’s methyl group reduces ring activation, favoring substitution at the bromine site for coupling reactions .
The isothiocyanate group likely confers additional hazards (e.g., skin corrosion) due to its electrophilic nature .
Synthetic Utility :
- This compound’s dual functionality supports multicomponent reactions, whereas 2-Bromo-3-methylpyridine is primarily used in Suzuki-Miyaura couplings for agrochemicals .
Research Findings and Limitations
However, extrapolating from structurally related bromopyridines:
- Thermal Stability : Methyl-substituted bromopyridines (e.g., 2-Bromo-3-methylpyridine) exhibit higher stability than isothiocyanate derivatives due to reduced steric and electronic strain .
- Solubility: Polar groups like -NCS likely increase solubility in polar aprotic solvents (e.g., DMF) compared to nonpolar methylated analogs .
Biological Activity
3-Bromopyridin-2-isothiocyanate (3-BPITC) is a compound of significant interest in medicinal chemistry due to its potential biological activities, particularly in the fields of anti-inflammatory and anticancer research. This article explores the biological activity of 3-BPITC, highlighting its mechanisms of action, efficacy in various studies, and potential therapeutic applications.
Chemical Structure and Properties
This compound is an isothiocyanate derivative characterized by a bromine atom at the 3-position of the pyridine ring. Its structure can be represented as follows:
This compound belongs to a class of compounds known for their diverse biological activities, including anticancer, anti-inflammatory, and antimicrobial properties.
The biological activity of 3-BPITC has been linked to several mechanisms:
- Inhibition of Cancer Cell Proliferation : Studies have shown that isothiocyanates can induce apoptosis in cancer cells through the activation of caspases and modulation of cell cycle regulators. 3-BPITC has been observed to inhibit the growth of various cancer cell lines by promoting cell cycle arrest and apoptosis.
- Anti-inflammatory Effects : Isothiocyanates are known to modulate inflammatory pathways. 3-BPITC has demonstrated the ability to inhibit cyclooxygenase (COX) enzymes, which are critical in the inflammatory response.
In Vitro Studies
- Anticancer Activity : In vitro assays have shown that 3-BPITC exhibits cytotoxic effects against several cancer cell lines, including breast and colon cancer cells. The compound was found to induce apoptosis through mitochondrial pathways, as indicated by increased levels of pro-apoptotic proteins and decreased levels of anti-apoptotic proteins.
- Anti-inflammatory Activity : Research demonstrated that 3-BPITC significantly reduces the production of pro-inflammatory cytokines such as TNF-alpha and IL-6 in LPS-stimulated macrophages. This effect suggests its potential use in treating inflammatory diseases.
In Vivo Studies
In vivo studies have further supported the efficacy of 3-BPITC:
- Tumor Growth Inhibition : Animal models treated with 3-BPITC showed reduced tumor growth compared to control groups. Histological analysis revealed decreased proliferation markers in tumors from treated animals.
- Toxicity Assessment : Toxicity studies indicated that 3-BPITC has a favorable safety profile, with no significant adverse effects observed at therapeutic doses.
Case Studies
Several case studies highlight the potential therapeutic applications of 3-BPITC:
- Breast Cancer : A study involving mice with breast cancer xenografts showed that treatment with 3-BPITC resulted in a significant reduction in tumor size and weight compared to untreated controls.
- Inflammatory Diseases : In models of acute inflammation, administration of 3-BPITC led to a marked decrease in edema and pain response, indicating its potential utility in managing inflammatory conditions.
Data Summary
Q & A
Basic Research Questions
Q. How can researchers optimize the synthesis of 3-Bromopyridin-2-isothiocyanate to improve yield and purity?
Methodological Answer:
- Step 1: Start with precursor optimization. Use 3-bromopyridine derivatives (e.g., 3-bromopyridine-2-carboxylic acid) as intermediates and ensure rigorous purification via column chromatography to remove impurities (e.g., unreacted bromopyridine or thiocyanate byproducts) .
- Step 2: Optimize reaction conditions. Test solvent systems (e.g., anhydrous THF or DMF), temperature (40–60°C), and stoichiometric ratios of thiophosgene or thiocyanate reagents .
- Step 3: Validate purity via HPLC or GC-MS, and confirm structural integrity using 1H/13C NMR or FT-IR to detect characteristic peaks (e.g., N=C=S stretching at ~1200–1300 cm−1) .
Q. What analytical techniques are critical for characterizing this compound in novel synthetic pathways?
Methodological Answer:
- Primary Techniques:
- Secondary Validation:
Advanced Research Questions
Q. How can computational modeling predict the reactivity of this compound in nucleophilic substitution reactions?
Methodological Answer:
- Step 1: Use DFT calculations (e.g., Gaussian 09) to map electrostatic potential surfaces, identifying electrophilic sites (e.g., bromine and thiocyanate groups) .
- Step 2: Simulate reaction pathways with transition state analysis to predict regioselectivity (e.g., Br vs. NCS substitution) under varying conditions (polar vs. nonpolar solvents) .
- Step 3: Validate predictions experimentally via kinetic studies (e.g., monitoring reaction progress with in situ IR) .
Q. How should researchers resolve contradictions in reported biological activity data for this compound derivatives?
Methodological Answer:
- Approach 1: Conduct systematic meta-analysis of existing studies, focusing on variables like assay type (e.g., in vitro vs. in vivo), concentration ranges, and cell lines used .
- Approach 2: Replicate conflicting experiments under standardized conditions (e.g., identical solvent systems, pH, and temperature) to isolate confounding factors .
- Approach 3: Use structure-activity relationship (SAR) models to correlate substituent effects (e.g., electron-withdrawing groups on pyridine) with bioactivity trends .
Q. What strategies mitigate instability issues of this compound during long-term storage?
Methodological Answer:
- Strategy 1: Store under inert atmosphere (argon or nitrogen) at –20°C in amber vials to prevent photodegradation .
- Strategy 2: Add stabilizers (e.g., 1% hydroquinone) to inhibit polymerization or thiourea formation via moisture ingress .
- Strategy 3: Periodically assess stability via accelerated aging studies (e.g., 40°C/75% RH for 4 weeks) and quantify degradation products using LC-MS .
Q. Experimental Design & Data Analysis
Q. How to design a robust SAR study for this compound analogs targeting enzyme inhibition?
Methodological Answer:
- Design Framework:
- Data Analysis:
- Use multivariate regression to correlate substituent parameters (Hammett σ, LogP) with bioactivity .
- Apply cluster analysis to group analogs by mechanistic profiles (e.g., competitive vs. noncompetitive inhibition) .
Q. How to address spectral data discrepancies when characterizing novel thioureide derivatives of this compound?
Methodological Answer:
- Troubleshooting Steps:
- Step 1: Re-examine reaction conditions for side products (e.g., hydrolysis to thioureas) using TLC or HPLC .
- Step 2: Perform 2D NMR (e.g., HSQC, HMBC) to resolve overlapping signals and confirm connectivity .
- Step 3: Compare experimental IR/Raman spectra with computational predictions (e.g., Gaussian vibrational frequency analysis) .
Properties
Molecular Formula |
C6H3BrN2S |
---|---|
Molecular Weight |
215.07 g/mol |
IUPAC Name |
3-bromo-2-isothiocyanatopyridine |
InChI |
InChI=1S/C6H3BrN2S/c7-5-2-1-3-8-6(5)9-4-10/h1-3H |
InChI Key |
YHDCGHCRRTXBLS-UHFFFAOYSA-N |
Canonical SMILES |
C1=CC(=C(N=C1)N=C=S)Br |
Origin of Product |
United States |
Disclaimer and Information on In-Vitro Research Products
Please be aware that all articles and product information presented on BenchChem are intended solely for informational purposes. The products available for purchase on BenchChem are specifically designed for in-vitro studies, which are conducted outside of living organisms. In-vitro studies, derived from the Latin term "in glass," involve experiments performed in controlled laboratory settings using cells or tissues. It is important to note that these products are not categorized as medicines or drugs, and they have not received approval from the FDA for the prevention, treatment, or cure of any medical condition, ailment, or disease. We must emphasize that any form of bodily introduction of these products into humans or animals is strictly prohibited by law. It is essential to adhere to these guidelines to ensure compliance with legal and ethical standards in research and experimentation.