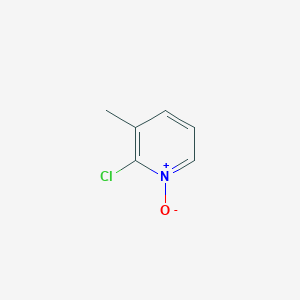
2-Chloro-3-methylpyridine 1-oxide
Overview
Description
2-Chloro-3-methylpyridine 1-oxide (CAS: 91668-83-6) is a halogenated pyridine derivative characterized by a chlorine atom at the 2-position, a methyl group at the 3-position, and an oxygen atom at the 1-position (N-oxide). This compound is commercially available with a purity of 98% . Its structure combines electron-withdrawing (chlorine) and electron-donating (methyl) substituents, which influence its reactivity and physicochemical properties. Pyridine N-oxides are widely utilized in pharmaceuticals, agrochemicals, and organic synthesis due to their enhanced solubility and ability to participate in unique reaction pathways compared to non-oxidized pyridines.
Preparation Methods
Synthetic Routes and Reaction Conditions:
One of the common methods for synthesizing 2-Chloro-3-methylpyridine 1-oxide involves the oxidation of 2-Chloro-3-methylpyridine. This can be achieved using oxidizing agents such as hydrogen peroxide or peracids under controlled conditions . Another method involves the reaction of 3-methylpyridine-1-oxide with phosgene in the presence of trimethylamine, followed by purification steps .
Industrial Production Methods:
In industrial settings, the production of this compound often involves large-scale oxidation processes using catalytic systems to ensure high yield and purity. The reaction conditions are optimized to maintain the stability of the compound and minimize by-products .
Chemical Reactions Analysis
Types of Reactions:
Oxidation: 2-Chloro-3-methylpyridine 1-oxide can undergo further oxidation to form more complex derivatives.
Reduction: It can be reduced back to 2-Chloro-3-methylpyridine using reducing agents like lithium aluminum hydride.
Substitution: The chlorine atom in the compound can be substituted with other functional groups through nucleophilic substitution reactions.
Common Reagents and Conditions:
Oxidation: Hydrogen peroxide, peracids.
Reduction: Lithium aluminum hydride, sodium borohydride.
Substitution: Nucleophiles such as amines, thiols under basic conditions.
Major Products Formed:
Oxidation: Higher oxidation state derivatives.
Reduction: 2-Chloro-3-methylpyridine.
Substitution: Various substituted pyridine derivatives depending on the nucleophile used.
Scientific Research Applications
Intermediate for Drug Synthesis
2-Chloro-3-methylpyridine 1-oxide serves as an important intermediate in the synthesis of various pharmaceuticals. It is utilized in the production of medications such as fluazifop and nicosulfuron, which are herbicides that target specific weed species while minimizing harm to crops . The compound's ability to act as a building block for more complex molecules makes it valuable in medicinal chemistry.
Case Study: Fluazifop Production
Fluazifop, a systemic herbicide, is synthesized using 2-chloro-3-methylpyridine as a key intermediate. The synthesis involves several steps where the chlorinated pyridine undergoes further transformations to yield the final herbicidal product. Studies have shown that this pathway not only enhances efficiency but also improves yield compared to traditional methods .
Insecticides
The compound is also a critical component in the formulation of neonicotinoid insecticides, including imidacloprid. These insecticides act as neurotoxins against pests, providing effective control while being less toxic to non-target organisms . The incorporation of 2-chloro-3-methylpyridine into these formulations has been linked with increased efficacy and reduced environmental impact.
Table 2: Agrochemical Applications
Agrochemical | Active Ingredient | Application |
---|---|---|
Fluazifop | Fluazifop-P-butyl | Herbicide for grass control |
Imidacloprid | Imidacloprid | Insecticide against sucking pests |
Bioconversion Studies
Recent research has explored the bioconversion of pyridine derivatives, including 2-chloro-3-methylpyridine, using microbial systems. For instance, studies utilizing Burkholderia sp. MAK1 have demonstrated the ability to hydroxylate pyridine derivatives effectively, suggesting potential pathways for biotransformation that could lead to novel compounds with enhanced biological activity .
Case Study: Microbial Transformation
In one study, the microbial transformation of pyridin-2-ones was investigated, revealing that specific strains could convert these substrates into hydroxylated products efficiently under optimized conditions (30 °C). This bioconversion not only highlights the versatility of 2-chloro-3-methylpyridine but also opens avenues for green chemistry applications in drug development .
Mechanism of Action
The mechanism of action of 2-Chloro-3-methylpyridine 1-oxide involves its interaction with various molecular targets. The N-oxide group can participate in redox reactions, influencing the compound’s reactivity and interaction with biological molecules. The chlorine atom can undergo substitution reactions, allowing the compound to form covalent bonds with target molecules .
Comparison with Similar Compounds
Comparison with Structurally Similar Compounds
Positional Isomers: Chloro-Methylpyridine 1-oxides
The position of substituents significantly affects the properties and reactivity of pyridine N-oxides. Key isomers include:
Functional Group Variations: Nitro and Bromo Derivatives
2-Chloro-3-methyl-4-nitropyridine 1-oxide (CAS: 60323-95-7)
This derivative features an additional nitro group at the 4-position. The nitro group increases electron deficiency, making the compound more reactive toward nucleophilic aromatic substitution.
2,4,6-Tribromopyridine 1-oxide (CAS: 170875-37-3)
With bromine atoms at the 2-, 4-, and 6-positions, this compound exhibits high density (2.54 g/cm³) and a low pKa (-2.36), reflecting strong electron-withdrawing effects. Its boiling point (427.4°C) and stability under heat contrast with chloro-methyl analogs, which are typically less thermally robust .
Key Insight : Halogen type (Cl vs. Br) and additional substituents (e.g., nitro) drastically alter reactivity and safety profiles. Bromine’s larger atomic radius increases steric bulk, while nitro groups enhance electrophilicity but require stringent handling protocols.
Cyanide-Mediated Reactions
3-Hydroxymethylpyridine 1-oxide reacts with methyl fluorosulfonate and cyanide to form 2,6-dicyano-3-methylpyridine, showcasing the versatility of N-oxides in generating nitrile derivatives . In contrast, this compound’s chlorine substituent may direct reactions toward displacement rather than rearrangement, as seen in its isomerization-resistant behavior.
Oxidation and Stability
Lepidine (4-methylquinoline) 1-oxide undergoes oxidation with SeO₂/peroxide, a reaction sensitive to solvent and peroxide ratios .
Biological Activity
2-Chloro-3-methylpyridine 1-oxide is a heterocyclic compound with significant biological activity, particularly in the fields of pharmacology and biochemistry. This compound, characterized by its molecular formula , has garnered attention due to its interactions with various biological targets, including enzymes and cellular pathways. This article explores the biological activity of this compound, including its mechanisms of action, biochemical properties, and relevant case studies.
The biological activity of this compound can be attributed to its ability to interact with several key enzymes and receptors:
- Cytochrome P450 Inhibition : Notably, this compound acts as an inhibitor of cytochrome P450 enzyme CYP1A2. This inhibition can significantly affect drug metabolism and pharmacokinetics, making it relevant in pharmacological studies.
- Enzyme Interactions : It has been shown to interact with various enzymes involved in metabolic pathways, influencing their activity and potentially leading to altered cellular functions.
Table 1: Summary of Biological Activities
Biological Activity | Target Enzyme/Pathway | Effect |
---|---|---|
Inhibition of CYP1A2 | Cytochrome P450 | Alters drug metabolism |
Interaction with Methane Monooxygenase | Enzymatic inhibition | Affects methane oxidation process |
Modulation of Metabolic Pathways | Various enzymes | Influences cellular metabolism |
The biochemical properties of this compound highlight its potential applications in medicinal chemistry:
- Oxidative Reactions : The N-oxide group allows the compound to participate in various oxidative reactions, acting both as an oxidizing agent and a substrate for further oxidation under specific conditions.
- Cellular Effects : Research indicates that this compound can influence cell signaling pathways, gene expression, and cellular metabolism. It has been observed to inhibit the growth of certain bacterial populations by interfering with their metabolic processes .
Case Studies
Several studies have documented the biological activity of this compound:
- Pharmacological Relevance : A study demonstrated that the inhibition of CYP1A2 by this compound could lead to significant changes in the pharmacokinetics of co-administered drugs. This finding underscores the importance of understanding drug interactions involving this compound in clinical settings.
- Antimicrobial Activity : Another investigation reported that this compound exhibited antimicrobial properties against specific bacterial strains. This effect was attributed to its ability to disrupt metabolic pathways critical for bacterial growth .
Molecular Mechanisms
At the molecular level, the actions of this compound involve specific binding interactions with biomolecules:
- Covalent Bond Formation : The compound is known to form covalent bonds with active sites on target enzymes, effectively inhibiting their normal function. This mechanism is particularly relevant in its interaction with methane monooxygenase and ammonia monooxygenase.
Q & A
Basic Research Questions
Q. What are the common synthetic routes for preparing 2-chloro-3-methylpyridine 1-oxide, and what factors influence reaction efficiency?
- Methodological Answer : The compound is typically synthesized via alkylation of pyridine 1-oxides using methyl fluorosulfonate, followed by chlorination. For example, 3-hydroxymethylpyridine 1-oxide reacts with methyl fluorosulfonate to form a quaternary salt, which is then treated with cyanide to yield nitrile derivatives. Reaction efficiency depends on the alkylating agent's strength, solvent polarity, and temperature control to avoid side reactions . Chlorination steps may require optimization of stoichiometry (e.g., SOCl₂ or PCl₅) and reaction time to minimize decomposition .
Q. How can spectroscopic techniques (NMR, FT-IR) be utilized to confirm the structure of this compound?
- Methodological Answer :
- ¹H/¹³C NMR : The methyl group at position 3 appears as a singlet (~δ 2.5 ppm for ¹H; ~δ 20–25 ppm for ¹³C). The N-oxide moiety deshields adjacent protons, shifting aromatic protons downfield (δ 7.5–8.5 ppm) .
- FT-IR : Key peaks include N-O stretch (~1250–1350 cm⁻¹) and C-Cl stretch (~600–800 cm⁻¹). Absence of hydroxyl stretches confirms successful dehydration in synthesis .
Q. What purification strategies are effective for isolating this compound from reaction mixtures?
- Methodological Answer : Column chromatography (silica gel, eluting with ethyl acetate/hexane) effectively separates polar N-oxide derivatives. Recrystallization from ethanol/water mixtures improves purity, leveraging differential solubility. Monitoring by TLC (Rf ~0.3–0.4 in 1:1 EtOAc/hexane) ensures fraction collection accuracy .
Advanced Research Questions
Q. What mechanistic insights explain the regioselectivity of alkylation in pyridine 1-oxide derivatives like this compound?
- Methodological Answer : Methyl fluorosulfonate preferentially alkylates the oxygen atom in pyridine 1-oxides due to its strong electrophilicity, forming a 1-alkoxypyridinium intermediate. Cyanide attack occurs at the α- and β-positions relative to the N-oxide, driven by charge distribution and steric hindrance from the methyl group. Computational studies (e.g., DFT) can model transition states to predict regioselectivity .
Q. How can computational methods (DFT, NBO analysis) complement experimental data in analyzing the electronic structure of this compound?
- Methodological Answer :
- DFT : Optimize molecular geometry using B3LYP/6-311++G(d,p) to calculate bond lengths and angles. Compare with X-ray crystallography data (if available) to validate accuracy.
- NBO Analysis : Quantify hyperconjugative interactions (e.g., n→σ* for N-O) to explain stabilization effects. HOMO-LUMO gaps (~5–6 eV) predict reactivity toward electrophiles/nucleophiles .
Q. What role does this compound play in synthesizing bioactive molecules or pharmaceutical intermediates?
- Methodological Answer : The compound serves as a precursor for fusaric acid analogs and p38 MAP kinase inhibitors. For example, nitrile groups introduced via cyanide substitution can be hydrolyzed to carboxylic acids for drug candidates. Its chlorine atom enables cross-coupling reactions (e.g., Suzuki-Miyaura) to build heterocyclic scaffolds .
Q. How should researchers address contradictions in reported reaction yields for this compound synthesis under similar conditions?
- Methodological Answer : Variability often stems from trace moisture (deactivates alkylating agents) or impurities in starting materials. Replicate reactions under inert atmospheres (argon/glovebox) and characterize intermediates (e.g., quaternary salts via ¹H NMR) to identify bottlenecks. Statistical design of experiments (DoE) can optimize parameters like temperature and reagent stoichiometry .
Q. What are the stability and storage requirements for this compound to prevent decomposition?
- Methodological Answer : Store at 2–8°C in amber vials under inert gas (N₂/Ar) to avoid hydrolysis of the N-oxide group. Monitor purity via HPLC (C18 column, acetonitrile/water mobile phase) periodically. Decomposition products (e.g., pyridines) can be identified by GC-MS .
Q. Can this compound participate in electrochemical C–H functionalization reactions?
- Methodological Answer : Yes, the N-oxide group enhances substrate solubility in polar solvents (e.g., DMF) and stabilizes radical intermediates during electro-reductive cross-electrophile coupling. Use a divided cell with a Pt cathode and sacrificial anode (e.g., Mg) at −1.5 V to achieve C–N bond formation .
Q. What challenges arise in functionalizing this compound while preserving the N-oxide group?
- Methodological Answer : The N-oxide is sensitive to reducing agents and strong acids. Use mild conditions (e.g., Pd-catalyzed couplings at ≤80°C) and avoid protic solvents. Protecting groups (e.g., acetyl for amines) may be required for multi-step syntheses .
Properties
IUPAC Name |
2-chloro-3-methyl-1-oxidopyridin-1-ium | |
---|---|---|
Source | PubChem | |
URL | https://pubchem.ncbi.nlm.nih.gov | |
Description | Data deposited in or computed by PubChem | |
InChI |
InChI=1S/C6H6ClNO/c1-5-3-2-4-8(9)6(5)7/h2-4H,1H3 | |
Source | PubChem | |
URL | https://pubchem.ncbi.nlm.nih.gov | |
Description | Data deposited in or computed by PubChem | |
InChI Key |
ZGHMADNNOPPAJO-UHFFFAOYSA-N | |
Source | PubChem | |
URL | https://pubchem.ncbi.nlm.nih.gov | |
Description | Data deposited in or computed by PubChem | |
Canonical SMILES |
CC1=C([N+](=CC=C1)[O-])Cl | |
Source | PubChem | |
URL | https://pubchem.ncbi.nlm.nih.gov | |
Description | Data deposited in or computed by PubChem | |
Molecular Formula |
C6H6ClNO | |
Source | PubChem | |
URL | https://pubchem.ncbi.nlm.nih.gov | |
Description | Data deposited in or computed by PubChem | |
DSSTOX Substance ID |
DTXSID70540605 | |
Record name | 2-Chloro-3-methyl-1-oxo-1lambda~5~-pyridine | |
Source | EPA DSSTox | |
URL | https://comptox.epa.gov/dashboard/DTXSID70540605 | |
Description | DSSTox provides a high quality public chemistry resource for supporting improved predictive toxicology. | |
Molecular Weight |
143.57 g/mol | |
Source | PubChem | |
URL | https://pubchem.ncbi.nlm.nih.gov | |
Description | Data deposited in or computed by PubChem | |
CAS No. |
91668-83-6 | |
Record name | 2-Chloro-3-methyl-1-oxo-1lambda~5~-pyridine | |
Source | EPA DSSTox | |
URL | https://comptox.epa.gov/dashboard/DTXSID70540605 | |
Description | DSSTox provides a high quality public chemistry resource for supporting improved predictive toxicology. | |
Synthesis routes and methods I
Procedure details
Synthesis routes and methods II
Procedure details
Synthesis routes and methods III
Procedure details
Synthesis routes and methods IV
Procedure details
Retrosynthesis Analysis
AI-Powered Synthesis Planning: Our tool employs the Template_relevance Pistachio, Template_relevance Bkms_metabolic, Template_relevance Pistachio_ringbreaker, Template_relevance Reaxys, Template_relevance Reaxys_biocatalysis model, leveraging a vast database of chemical reactions to predict feasible synthetic routes.
One-Step Synthesis Focus: Specifically designed for one-step synthesis, it provides concise and direct routes for your target compounds, streamlining the synthesis process.
Accurate Predictions: Utilizing the extensive PISTACHIO, BKMS_METABOLIC, PISTACHIO_RINGBREAKER, REAXYS, REAXYS_BIOCATALYSIS database, our tool offers high-accuracy predictions, reflecting the latest in chemical research and data.
Strategy Settings
Precursor scoring | Relevance Heuristic |
---|---|
Min. plausibility | 0.01 |
Model | Template_relevance |
Template Set | Pistachio/Bkms_metabolic/Pistachio_ringbreaker/Reaxys/Reaxys_biocatalysis |
Top-N result to add to graph | 6 |
Feasible Synthetic Routes
Disclaimer and Information on In-Vitro Research Products
Please be aware that all articles and product information presented on BenchChem are intended solely for informational purposes. The products available for purchase on BenchChem are specifically designed for in-vitro studies, which are conducted outside of living organisms. In-vitro studies, derived from the Latin term "in glass," involve experiments performed in controlled laboratory settings using cells or tissues. It is important to note that these products are not categorized as medicines or drugs, and they have not received approval from the FDA for the prevention, treatment, or cure of any medical condition, ailment, or disease. We must emphasize that any form of bodily introduction of these products into humans or animals is strictly prohibited by law. It is essential to adhere to these guidelines to ensure compliance with legal and ethical standards in research and experimentation.