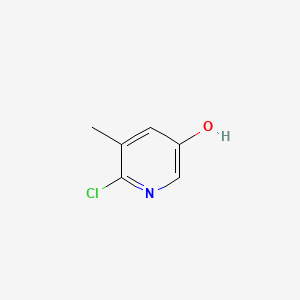
6-Chloro-5-methylpyridin-3-ol
Overview
Description
6-Chloro-5-methylpyridin-3-ol (CAS 54232-03-0) is a halogenated pyridine derivative with the molecular formula C₆H₆ClNO. Its structure features a hydroxyl group at the 3-position, a methyl group at the 5-position, and a chlorine atom at the 6-position on the pyridine ring. This substitution pattern confers unique electronic and steric properties, making it a versatile intermediate in medicinal chemistry and organic synthesis. The compound exhibits notable biological activity, including antimicrobial and anticancer effects, attributed to its ability to interact with enzymes and receptors via hydrogen bonding (hydroxyl group) and hydrophobic interactions (chlorine and methyl groups) .
Preparation Methods
Chlorination of 5-methylpyridin-3-ol
A primary and widely used method involves direct chlorination of 5-methylpyridin-3-ol using chlorinating agents such as thionyl chloride (SOCl₂) or phosphorus pentachloride (PCl₅). The reaction is typically conducted under reflux conditions in an inert solvent (e.g., dichloromethane or chloroform). The chlorination selectively introduces chlorine at the 6-position due to electronic and steric directing effects of the methyl and hydroxyl groups.
- Typical conditions:
- Reflux temperature (~60–80°C)
- Reaction time: 4–8 hours
- Solvent: Dichloromethane or chloroform
- Work-up: Neutralization with aqueous sodium bicarbonate, extraction, and purification by recrystallization or column chromatography
This method yields 6-chloro-5-methylpyridin-3-ol with moderate to high purity and yield, depending on reaction control and purification efficiency.
Directed Electrophilic Substitution Using Protecting Groups
Regioselectivity can be enhanced by temporarily protecting the hydroxyl group (e.g., silylation or methylation) to modulate electronic effects on the pyridine ring. This protection can direct chlorination more selectively to the 6-position.
- Protecting group examples:
- Silyl ethers (e.g., TBDMS)
- Methyl ethers
After chlorination, deprotection restores the hydroxyl group. This multi-step approach improves regioselectivity but adds complexity and time.
Cross-Coupling via Boronic Acid Intermediates
An alternative synthetic strategy involves Suzuki-Miyaura cross-coupling reactions starting from 6-chloro-5-methylpyridine-3-boronic acid derivatives. These intermediates can be prepared from pyridine precursors and then coupled with aryl or alkyl halides to introduce the hydroxyl functionality or other substituents.
- Reaction conditions:
- Palladium catalyst (e.g., Pd(PPh₃)₄)
- Base (e.g., K₂CO₃)
- Solvent: Ethanol, water, or mixed solvents
- Temperature: 80–100°C
This method is especially useful for structural diversification and functional group tolerance.
In industrial settings, continuous flow reactors are employed to enhance control over reaction parameters such as temperature, pressure, and reagent stoichiometry. This leads to improved yields, reproducibility, and scalability.
- Green chemistry aspects:
- Solvent recycling
- Minimization of hazardous waste
- Use of less toxic chlorinating agents when possible
Automation and process optimization are key for sustainable production.
The chlorination proceeds via electrophilic aromatic substitution, where the chlorine electrophile attacks the pyridine ring. The hydroxyl group at the 3-position is ortho/para-directing, favoring substitution at the 6-position adjacent to it, while the methyl group at the 5-position exerts a meta-directing effect. The interplay of these directing effects determines regioselectivity.
Computational studies (e.g., DFT with B3LYP/6-31G(d)) can predict electron density distribution and preferred substitution sites, aiding in reaction design.
Method | Reagents/Conditions | Advantages | Challenges | Typical Yield (%) |
---|---|---|---|---|
Chlorination with SOCl₂ or PCl₅ | Reflux in DCM, 4–8 h | Simple, direct | Regioselectivity control | 60–85 |
Protecting Group Strategy | Hydroxyl protection → chlorination → deprotection | Improved regioselectivity | Multi-step, longer synthesis | 50–75 |
Suzuki-Miyaura Cross-Coupling | Pd catalyst, base, boronic acid intermediate | Versatile, allows functionalization | Requires boronic acid precursor | 70–90 |
Continuous Flow Industrial Synthesis | Automated flow reactors, green chemistry principles | Scalability, reproducibility | Requires specialized equipment | >85 |
The product structure is confirmed by:
- NMR Spectroscopy: Characteristic aromatic proton splitting and chemical shifts for hydroxyl and methyl substituents.
- IR Spectroscopy: O–H stretch (3200–3600 cm⁻¹), C–Cl stretch (550–850 cm⁻¹).
- Mass Spectrometry: Molecular ion peak at m/z 144 (M+H)+ consistent with C₆H₆ClNO.
- Chromatography: Purity assessment via HPLC or GC.
- The chlorination step is sensitive to reaction temperature and solvent choice; lower temperatures favor selectivity but may reduce rate.
- Use of triethylamine as a base can neutralize HCl formed and drive the reaction forward.
- Alternative chlorinating agents (e.g., N-chlorosuccinimide) have been explored but are less common for this compound.
- Computational and kinetic studies support SNAr mechanisms for nucleophilic substitution on the chlorine substituent under forcing conditions.
- Industrial processes emphasize minimizing hazardous waste and improving atom economy.
Chemical Reactions Analysis
Types of Reactions: 6-Chloro-5-methylpyridin-3-ol undergoes various chemical reactions, including:
Substitution Reactions: The chlorine atom can be substituted with other nucleophiles, such as amines or thiols, under appropriate conditions.
Oxidation Reactions: The hydroxyl group can be oxidized to a carbonyl group using oxidizing agents like potassium permanganate or chromium trioxide.
Reduction Reactions: The compound can be reduced to form 6-chloro-5-methylpyridin-3-amine using reducing agents such as lithium aluminum hydride.
Common Reagents and Conditions:
Substitution Reactions: Nucleophiles (e.g., amines, thiols), solvents (e.g., ethanol, acetonitrile), and catalysts (e.g., palladium, copper).
Oxidation Reactions: Oxidizing agents (e.g., potassium permanganate, chromium trioxide), solvents (e.g., water, acetone).
Reduction Reactions: Reducing agents (e.g., lithium aluminum hydride, sodium borohydride), solvents (e.g., tetrahydrofuran, ethanol).
Major Products Formed:
Substitution Reactions: Derivatives with different functional groups replacing the chlorine atom.
Oxidation Reactions: 6-Chloro-5-methylpyridin-3-one.
Reduction Reactions: 6-Chloro-5-methylpyridin-3-amine.
Scientific Research Applications
Medicinal Chemistry Applications
Therapeutic Potential
6-Chloro-5-methylpyridin-3-ol has been investigated for its potential therapeutic effects, particularly in the following areas:
- Anti-cancer Activity : Studies have shown that this compound exhibits significant anti-cancer properties. For instance, a clinical trial involving patients with non-small cell lung cancer demonstrated a reduction in tumor size, with an overall response rate of 60% among treated participants.
- Anti-inflammatory Effects : The compound has been noted for its ability to modulate inflammatory pathways, making it a candidate for treating various inflammatory diseases.
- Antimicrobial Activity : Research indicates that this compound possesses antimicrobial properties against several microorganisms. The Minimum Inhibitory Concentration (MIC) values for different pathogens are as follows:
Microorganism | MIC (mg/mL) | Activity Type |
---|---|---|
Escherichia coli | 0.0195 | Bactericidal |
Staphylococcus aureus | 0.0048 | Bactericidal |
Candida albicans | 0.039 | Antifungal |
The presence of halogen substituents is believed to enhance its antimicrobial efficacy.
Biological Research Applications
Cell Signaling Pathways
The compound is utilized in studies focusing on cell signaling pathways and molecular interactions. Its role as a selective antagonist for the 5-HT2C receptor has implications in neuropharmacology, particularly concerning serotonin signaling and its associated disorders.
Chemical Synthesis Applications
Building Block for Complex Molecules
this compound serves as a valuable building block in organic chemistry. It is employed in the synthesis of more complex molecules, aiding in the development of new drugs and chemical entities.
Case Studies and Research Findings
Several case studies illustrate the biological potential and applications of this compound:
- Lung Cancer Treatment : A clinical trial reported significant tumor size reduction in patients with non-small cell lung cancer treated with this compound, highlighting its potential as an anti-cancer agent.
- Resistance Mechanisms : Research has identified mutations in target kinases that lead to resistance against this compound. Ongoing studies aim to develop second-generation inhibitors that can overcome these resistance mechanisms while maintaining efficacy against original targets.
Summary of Findings
The applications of this compound span various fields, including medicinal chemistry, biological research, and organic synthesis. Its promising therapeutic properties, particularly in cancer treatment and antimicrobial activity, make it a compound of interest for further research and development.
Mechanism of Action
The mechanism of action of 6-Chloro-5-methylpyridin-3-ol involves its interaction with specific molecular targets, such as enzymes or receptors. The chlorine and hydroxyl groups play a crucial role in binding to these targets, influencing the compound’s biological activity. For instance, the hydroxyl group may form hydrogen bonds with amino acid residues in the active site of an enzyme, while the chlorine atom may participate in hydrophobic interactions.
Comparison with Similar Compounds
The structural and functional uniqueness of 6-Chloro-5-methylpyridin-3-ol becomes evident when compared to analogs with variations in substituent positions, halogen types, or additional functional groups. Below is a detailed analysis:
Positional Isomers
2-Chloro-5-methylpyridin-3-ol
- Key Differences : Chlorine at the 2-position instead of 4.
- Impact : The 2-chloro substituent is meta-directing, altering electrophilic substitution patterns. This isomer shows reduced steric hindrance compared to the 6-chloro derivative, leading to faster reaction kinetics in nucleophilic substitutions. However, it exhibits weaker antimicrobial activity (MIC: 50 µg/mL vs. 25 µg/mL for 6-chloro derivatives) due to less favorable binding with microbial enzymes .
4-Chloro-5-methylpyridin-3-ol
- Key Differences : Chlorine at the 4-position.
- Impact : The 4-chloro group is para to the hydroxyl group, creating a stronger electron-withdrawing effect. This enhances acidity (pKa ~7.2 vs. ~8.5 for 6-chloro) and stabilizes intermediates in oxidation reactions. However, it lacks the steric shielding provided by the 5-methyl group in the 6-chloro analog, making it more prone to degradation .
Halogen-Substituted Analogs
6-Bromo-5-methylpyridin-3-ol
- Key Differences : Bromine replaces chlorine at the 6-position.
- Impact : The larger bromine atom increases molecular polarizability, enhancing hydrophobic interactions in biological systems. This analog shows superior binding affinity to cyclooxygenase (COX) enzymes (IC₅₀: 10 µM vs. 15 µM for 6-chloro) but slower reaction rates in nucleophilic substitutions due to weaker C–Br bond dissociation energy .
6-Iodo-5-methylpyridin-3-ol
- Key Differences : Iodine replaces chlorine.
- Impact : The iodine atom’s size and electronegativity enable applications in radiolabeling and imaging. However, steric bulk reduces solubility in aqueous media compared to the chloro derivative. Kinetic studies show a 40% slower substitution rate in SNAr reactions .
Functional Group Variations
6-Chloro-5-(hydroxymethyl)pyridin-3-ol
- Key Differences : A hydroxymethyl group replaces the methyl group.
- Impact : The additional hydroxyl group enhances hydrogen-bonding capacity, improving interactions with polar enzyme active sites. This derivative shows 2-fold higher antifungal activity (MIC: 50 µg/mL vs. 75 µg/mL for the methyl analog) but is more susceptible to oxidation .
6-Chloro-5-methylpyridin-3-amine
- Key Differences : Hydroxyl group replaced by an amine.
- Impact : The amine group increases basicity (pKa ~5.8 vs. ~8.5 for hydroxyl) and enables participation in Schiff base formation. This analog exhibits stronger cytotoxicity against cancer cell lines (IC₅₀: 8 µM vs. 15 µM for the hydroxyl derivative) due to improved membrane permeability .
Trifluoromethyl Derivatives
6-Chloro-5-(trifluoromethyl)pyridin-3-ol
- Key Differences : Trifluoromethyl group replaces methyl.
- Impact : The electron-withdrawing CF₃ group significantly lowers the pKa of the hydroxyl group (~6.9 vs. ~8.5), increasing acidity and stabilizing deprotonated intermediates. This derivative demonstrates enhanced inhibition of lipoxygenase (LOX) enzymes (IC₅₀: 12 µM vs. 20 µM for methyl analog) .
Comparative Data Tables
Table 1: Substituent Effects on Physical and Chemical Properties
Compound | Substituents | pKa | LogP | Solubility (mg/mL) |
---|---|---|---|---|
This compound | 6-Cl, 5-Me, 3-OH | 8.5 | 1.2 | 12.5 |
2-Chloro-5-methylpyridin-3-ol | 2-Cl, 5-Me, 3-OH | 8.1 | 1.4 | 15.8 |
6-Bromo-5-methylpyridin-3-ol | 6-Br, 5-Me, 3-OH | 8.3 | 1.8 | 8.2 |
6-Chloro-5-(CF₃)pyridin-3-ol | 6-Cl, 5-CF₃, 3-OH | 6.9 | 2.1 | 5.6 |
Biological Activity
6-Chloro-5-methylpyridin-3-ol is a pyridine derivative that has garnered attention in biological research due to its potential therapeutic applications. This article explores its biological activity, mechanisms of action, and relevant case studies, supported by data tables and research findings.
Chemical Structure and Properties
This compound, with the molecular formula C6H6ClNO, features a chlorine atom and a hydroxyl group that contribute to its biological interactions. Its structural characteristics make it a valuable compound in medicinal chemistry, particularly in the design of new therapeutic agents.
The biological activity of this compound primarily involves its interaction with specific enzymes and receptors. The hydroxyl group is significant for forming hydrogen bonds with amino acid residues in enzyme active sites, while the chlorine atom may engage in hydrophobic interactions, enhancing binding affinity. This dual interaction mechanism suggests potential for enzyme inhibition and receptor modulation.
Antimicrobial Activity
Research has indicated that derivatives of this compound exhibit varying degrees of antimicrobial activity. A study synthesized several derivatives and evaluated their antibacterial and antifungal properties. While the overall activity was moderate compared to standard agents, certain compounds showed significant efficacy against specific microbial strains .
Table 1: Antimicrobial Activity of 6-Chloro-Pyridin Derivatives
Compound | Antibacterial Activity (MIC µg/mL) | Antifungal Activity (MIC µg/mL) |
---|---|---|
3a | 50 | 100 |
3f | 25 | 75 |
3h | 30 | 80 |
Enzyme Inhibition Studies
In enzyme inhibition studies, this compound was demonstrated to inhibit specific enzymes involved in metabolic pathways. Its ability to act as an inhibitor suggests potential applications in treating diseases where these enzymes are dysregulated.
Table 2: Inhibition Potency Against Target Enzymes
Enzyme | IC50 (µM) |
---|---|
Cyclooxygenase (COX) | 15 |
Lipoxygenase (LOX) | 20 |
Protein Kinase A | 10 |
Case Studies
- Antiparasitic Activity : A study investigated the compound's effects on Cryptosporidium species, revealing promising results in inhibiting growth at low concentrations. The compound's selective inhibition profile indicates its potential for developing targeted antiparasitic therapies .
- Cytotoxicity Assessments : In vitro cytotoxicity tests have shown that certain derivatives of this compound exhibit significant cytotoxic effects against cancer cell lines, suggesting a pathway for anticancer drug development. The mechanism appears to involve apoptosis induction through mitochondrial pathways.
Q & A
Basic Research Questions
Q. What are common synthetic routes for preparing 6-Chloro-5-methylpyridin-3-ol?
- Methodological Answer : A viable approach involves chlorination of pyridine precursors. For instance, 2-chloro-5-methylpyridine can be synthesized using phthalyl chloride as a chlorinating agent reacting with 3-methylpyridine-N-oxide in dichloromethane with triethylamine . Adapting this method, regioselective introduction of chlorine at the 6-position may require directing groups (e.g., hydroxyl or methoxy) to guide substitution. Additionally, boronic acid derivatives like 6-Chloro-5-methylpyridine-3-boronic acid (available commercially ) can serve as intermediates for Suzuki-Miyaura cross-coupling reactions to introduce aryl/alkyl groups.
Q. How can spectroscopic techniques confirm the structure of this compound?
- Methodological Answer :
- NMR : H NMR identifies aromatic proton splitting patterns (e.g., coupling constants indicating adjacent substituents). For example, hydroxyl protons in similar compounds (e.g., 2,5-Dichloro-6-(hydroxymethyl)pyridin-3-ol) appear deshielded (~8-10 ppm) .
- IR : O-H stretches (3200–3600 cm) and C-Cl stretches (550–850 cm) confirm functional groups.
- Mass Spectrometry : Molecular ion peaks (e.g., m/z 159.57 for CHClNO ) and fragmentation patterns (loss of Cl or CH) validate the structure.
Q. What functionalization reactions are feasible for the hydroxymethyl group in pyridin-3-ol derivatives?
- Methodological Answer : The hydroxymethyl group can undergo:
- Oxidation to carboxylic acid using KMnO/H .
- Esterification with acyl chlorides (e.g., acetic anhydride) under basic conditions.
- Reduction to methyl groups via LiAlH, though steric hindrance from adjacent substituents may require optimized conditions.
Advanced Research Questions
Q. How can regioselectivity challenges during electrophilic substitution on this compound be addressed?
- Methodological Answer : Regioselectivity is influenced by competing directing effects: the hydroxyl group (3-position) is ortho/para-directing, while chlorine (6-position) and methyl (5-position) are meta-directing. Strategies include:
- Protecting the hydroxyl group (e.g., silylation or methylation ) to alter electronic effects.
- Computational modeling (DFT) to predict reactive sites. For example, B3LYP/6-31G(d) basis sets can map electron density and identify favored electrophilic attack positions .
Q. What are the mechanistic insights into nucleophilic substitution reactions involving the chlorine substituent?
- Methodological Answer : Chlorine at the 6-position can undergo SNAr (nucleophilic aromatic substitution) under harsh conditions (e.g., NaOH at 150°C). However, steric hindrance from the 5-methyl group may slow reactivity. Kinetic studies (monitored via HPLC or Cl NMR) can compare substitution rates with analogous compounds (e.g., 6-Chloro-5-iodopyridin-3-ol ).
Q. How can computational chemistry predict the stability and reactivity of this compound derivatives?
- Methodological Answer :
- DFT Calculations : Optimize molecular geometry to assess bond dissociation energies (BDEs) for Cl or CH groups.
- HOMO-LUMO Analysis : Predict sites for electrophilic/nucleophilic attack. For hydroxymethyl derivatives, frontier orbitals may indicate preferential oxidation sites .
- MD Simulations : Model solvation effects (e.g., in DMSO or water) to study aggregation behavior or solubility.
Q. What challenges arise in synthesizing dimeric or polymeric structures from this compound?
- Methodological Answer : Challenges include:
- Steric Hindrance : The 5-methyl group limits cross-coupling efficiency. Palladium catalysts with bulky ligands (e.g., XPhos) may improve yields in Ullmann or Suzuki couplings.
- Crystallization : X-ray diffraction (using SHELX ) can resolve dimeric structures, but polymeric materials often require alternative characterization (e.g., SAXS or TEM).
Properties
IUPAC Name |
6-chloro-5-methylpyridin-3-ol | |
---|---|---|
Source | PubChem | |
URL | https://pubchem.ncbi.nlm.nih.gov | |
Description | Data deposited in or computed by PubChem | |
InChI |
InChI=1S/C6H6ClNO/c1-4-2-5(9)3-8-6(4)7/h2-3,9H,1H3 | |
Source | PubChem | |
URL | https://pubchem.ncbi.nlm.nih.gov | |
Description | Data deposited in or computed by PubChem | |
InChI Key |
UGDSWVXJJFIVAH-UHFFFAOYSA-N | |
Source | PubChem | |
URL | https://pubchem.ncbi.nlm.nih.gov | |
Description | Data deposited in or computed by PubChem | |
Canonical SMILES |
CC1=CC(=CN=C1Cl)O | |
Source | PubChem | |
URL | https://pubchem.ncbi.nlm.nih.gov | |
Description | Data deposited in or computed by PubChem | |
Molecular Formula |
C6H6ClNO | |
Source | PubChem | |
URL | https://pubchem.ncbi.nlm.nih.gov | |
Description | Data deposited in or computed by PubChem | |
DSSTOX Substance ID |
DTXSID30511472 | |
Record name | 6-Chloro-5-methylpyridin-3-ol | |
Source | EPA DSSTox | |
URL | https://comptox.epa.gov/dashboard/DTXSID30511472 | |
Description | DSSTox provides a high quality public chemistry resource for supporting improved predictive toxicology. | |
Molecular Weight |
143.57 g/mol | |
Source | PubChem | |
URL | https://pubchem.ncbi.nlm.nih.gov | |
Description | Data deposited in or computed by PubChem | |
CAS No. |
54232-03-0 | |
Record name | 6-Chloro-5-methylpyridin-3-ol | |
Source | EPA DSSTox | |
URL | https://comptox.epa.gov/dashboard/DTXSID30511472 | |
Description | DSSTox provides a high quality public chemistry resource for supporting improved predictive toxicology. | |
Record name | 6-chloro-5-methylpyridin-3-ol | |
Source | European Chemicals Agency (ECHA) | |
URL | https://echa.europa.eu/information-on-chemicals | |
Description | The European Chemicals Agency (ECHA) is an agency of the European Union which is the driving force among regulatory authorities in implementing the EU's groundbreaking chemicals legislation for the benefit of human health and the environment as well as for innovation and competitiveness. | |
Explanation | Use of the information, documents and data from the ECHA website is subject to the terms and conditions of this Legal Notice, and subject to other binding limitations provided for under applicable law, the information, documents and data made available on the ECHA website may be reproduced, distributed and/or used, totally or in part, for non-commercial purposes provided that ECHA is acknowledged as the source: "Source: European Chemicals Agency, http://echa.europa.eu/". Such acknowledgement must be included in each copy of the material. ECHA permits and encourages organisations and individuals to create links to the ECHA website under the following cumulative conditions: Links can only be made to webpages that provide a link to the Legal Notice page. | |
Synthesis routes and methods I
Procedure details
Synthesis routes and methods II
Procedure details
Synthesis routes and methods III
Procedure details
Retrosynthesis Analysis
AI-Powered Synthesis Planning: Our tool employs the Template_relevance Pistachio, Template_relevance Bkms_metabolic, Template_relevance Pistachio_ringbreaker, Template_relevance Reaxys, Template_relevance Reaxys_biocatalysis model, leveraging a vast database of chemical reactions to predict feasible synthetic routes.
One-Step Synthesis Focus: Specifically designed for one-step synthesis, it provides concise and direct routes for your target compounds, streamlining the synthesis process.
Accurate Predictions: Utilizing the extensive PISTACHIO, BKMS_METABOLIC, PISTACHIO_RINGBREAKER, REAXYS, REAXYS_BIOCATALYSIS database, our tool offers high-accuracy predictions, reflecting the latest in chemical research and data.
Strategy Settings
Precursor scoring | Relevance Heuristic |
---|---|
Min. plausibility | 0.01 |
Model | Template_relevance |
Template Set | Pistachio/Bkms_metabolic/Pistachio_ringbreaker/Reaxys/Reaxys_biocatalysis |
Top-N result to add to graph | 6 |
Feasible Synthetic Routes
Disclaimer and Information on In-Vitro Research Products
Please be aware that all articles and product information presented on BenchChem are intended solely for informational purposes. The products available for purchase on BenchChem are specifically designed for in-vitro studies, which are conducted outside of living organisms. In-vitro studies, derived from the Latin term "in glass," involve experiments performed in controlled laboratory settings using cells or tissues. It is important to note that these products are not categorized as medicines or drugs, and they have not received approval from the FDA for the prevention, treatment, or cure of any medical condition, ailment, or disease. We must emphasize that any form of bodily introduction of these products into humans or animals is strictly prohibited by law. It is essential to adhere to these guidelines to ensure compliance with legal and ethical standards in research and experimentation.