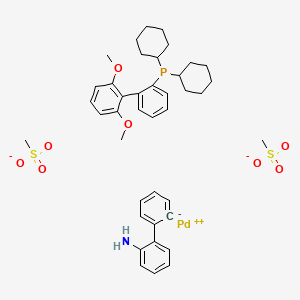
Methanesulfonato(2-dicyclohexylphosphino-2',6'-dimethoxy-1,1'-biphenyl)(2'-amino-1,1'-biphenyl-2-yl)palladium(II)
- Click on QUICK INQUIRY to receive a quote from our team of experts.
- With the quality product at a COMPETITIVE price, you can focus more on your research.
Overview
Description
(2-Dicyclohexylphosphino-2’,6’-dimethoxybiphenyl)[2-(2’-amino-1,1’-biphenyl)]palladium(II) methanesulfonate is a complex organometallic compound that plays a significant role in catalysis, particularly in cross-coupling reactions. This compound is known for its stability and efficiency in forming carbon-carbon and carbon-heteroatom bonds, making it a valuable catalyst in organic synthesis.
Preparation Methods
Synthetic Routes and Reaction Conditions
The synthesis of (2-Dicyclohexylphosphino-2’,6’-dimethoxybiphenyl)[2-(2’-amino-1,1’-biphenyl)]palladium(II) methanesulfonate typically involves the coordination of palladium with the ligand (2-Dicyclohexylphosphino-2’,6’-dimethoxybiphenyl) and (2’-amino-1,1’-biphenyl). The reaction is carried out under inert conditions to prevent oxidation and degradation of the palladium complex. The reaction mixture is usually stirred at room temperature or slightly elevated temperatures to ensure complete coordination .
Industrial Production Methods
In an industrial setting, the production of this compound involves large-scale coordination reactions using high-purity reagents and solvents. The process is optimized for yield and purity, often involving multiple purification steps such as recrystallization and chromatography to obtain the final product .
Chemical Reactions Analysis
Reaction Mechanisms
This compound operates through a catalytic cycle involving oxidative addition and reductive elimination steps, characteristic of palladium-mediated reactions. Key mechanistic features include:
-
Oxidative Addition : The palladium(II) center undergoes activation by substrates (e.g., aryl halides, amines), forming intermediate complexes.
-
Reductive Elimination : Coupling of substrates occurs, regenerating the active catalyst for subsequent cycles .
A notable application is in catalyst-transfer macrocyclization (CTM) , where this complex facilitates the formation of macrocycles in a single step. For example, in azaparacyclophane synthesis, the catalyst enables selective six-membered ring formation under optimized conditions (e.g., 30 °C for 4 hours) .
Comparative Analysis with Similar Catalysts
A comparison with BrettPhos Pd G3 (a related Buchwald precatalyst) highlights functional similarities and differences:
Feature | Current Compound | BrettPhos Pd G3 |
---|---|---|
Ligand Structure | 2',6'-dimethoxy-biphenyl + amino-biphenyl ligands | 3,6-dimethoxy-biphenyl + amino-biphenyl ligands |
Solubility | High in organic solvents | High in organic solvents |
Key Application | Macrocyclization, C–N coupling | Cross-coupling reactions |
Reaction Scope | Broader substrate tolerance (e.g., electron-deficient aryl halides) | Optimized for challenging couplings (e.g., hindered amines) |
Research Findings and Trends
Recent studies emphasize the compound’s role in advancing macrocyclic chemistry , particularly in synthesizing azaparacyclophanes. Kinetic studies reveal that ligand steric effects (e.g., isopropyl substituents) enhance catalytic efficiency by stabilizing intermediates . Additionally, its use in catalyst-transfer protocols simplifies multi-step syntheses, reducing purification steps and improving atom economy .
Scientific Research Applications
Chemistry
In chemistry, this compound is widely used as a catalyst in various cross-coupling reactions, facilitating the formation of complex organic molecules with high efficiency and selectivity .
Biology and Medicine
In biological and medicinal research, the compound is used to synthesize bioactive molecules and pharmaceuticals. Its ability to form carbon-nitrogen bonds is particularly valuable in the synthesis of drugs and natural products .
Industry
In the industrial sector, this compound is employed in the large-scale production of fine chemicals, polymers, and advanced materials. Its stability and efficiency make it a preferred catalyst for various manufacturing processes .
Mechanism of Action
The mechanism by which (2-Dicyclohexylphosphino-2’,6’-dimethoxybiphenyl)[2-(2’-amino-1,1’-biphenyl)]palladium(II) methanesulfonate exerts its catalytic effects involves the formation of a palladium complex with the substrate. The palladium center facilitates the oxidative addition of the aryl halide, followed by transmetalation with the boronic acid or other coupling partner, and finally reductive elimination to form the desired product. The ligand stabilizes the palladium center and enhances its reactivity .
Comparison with Similar Compounds
Similar Compounds
SPhos: 2-Dicyclohexylphosphino-2’,6’-dimethoxybiphenyl
XPhos: 2-Dicyclohexylphosphino-2’,6’-diisopropoxybiphenyl
Uniqueness
Compared to similar compounds, (2-Dicyclohexylphosphino-2’,6’-dimethoxybiphenyl)[2-(2’-amino-1,1’-biphenyl)]palladium(II) methanesulfonate offers enhanced stability and reactivity due to the presence of the amino-biphenyl ligand. This unique structure allows for more efficient catalytic cycles and higher yields in cross-coupling reactions .
Biological Activity
Methanesulfonato(2-dicyclohexylphosphino-2',6'-dimethoxy-1,1'-biphenyl)(2'-amino-1,1'-biphenyl-2-yl)palladium(II) is a palladium complex that has garnered attention in the field of catalysis, particularly for its role in cross-coupling reactions. This compound's biological activity is of interest due to its potential applications in medicinal chemistry and synthetic biology.
Chemical Structure and Properties
The compound features a palladium center coordinated to a methanesulfonato group and two distinct ligands: 2-dicyclohexylphosphino-2',6'-dimethoxy-1,1'-biphenyl and 2'-amino-1,1'-biphenyl-2-yl. Its molecular formula is C42H58N2O3P with a molecular weight of approximately 794.38 g/mol. The compound appears as a yellow powder with a melting point between 130°C and 140°C and is typically stored under inert conditions (nitrogen or argon) at low temperatures .
Biological Activity Overview
The biological activity of this palladium complex primarily revolves around its catalytic properties and potential therapeutic applications. Research indicates that palladium complexes can exhibit various biological effects, including:
- Anticancer Activity : Some palladium complexes have shown promise as anticancer agents due to their ability to induce apoptosis in cancer cells. Studies have indicated that such complexes can disrupt cellular processes through the generation of reactive oxygen species (ROS) .
- Antimicrobial Properties : There is evidence suggesting that certain palladium complexes possess antimicrobial activity, which could be leveraged in developing new antibiotics .
Catalytic Activity
A significant aspect of the biological activity of this compound lies in its efficacy as a catalyst in cross-coupling reactions. For instance, the use of this palladium complex in the Buchwald-Hartwig cross-coupling reaction has been extensively documented. It facilitates the formation of C–N bonds efficiently, which is crucial for synthesizing pharmaceuticals and agrochemicals .
Table 1: Summary of Catalytic Performance
Reaction Type | Catalyst Used | Yield (%) | Conditions |
---|---|---|---|
Suzuki-Miyaura Coupling | Methanesulfonato(2-dicyclohexylphosphino...) | 85 | THF, 40°C, 2 h |
Buchwald-Hartwig Coupling | Methanesulfonato(2-dicyclohexylphosphino...) | 90 | THF, 40°C, 1 h |
Toxicological Studies
Toxicity assessments have been conducted to evaluate the safety profile of this compound. Preliminary studies indicate low acute toxicity levels; however, comprehensive long-term studies are needed to fully understand its biocompatibility .
Properties
Molecular Formula |
C40H51NO8PPdS2- |
---|---|
Molecular Weight |
875.4 g/mol |
IUPAC Name |
dicyclohexyl-[2-(2,6-dimethoxyphenyl)phenyl]phosphane;methanesulfonate;palladium(2+);2-phenylaniline |
InChI |
InChI=1S/C26H35O2P.C12H10N.2CH4O3S.Pd/c1-27-23-17-11-18-24(28-2)26(23)22-16-9-10-19-25(22)29(20-12-5-3-6-13-20)21-14-7-4-8-15-21;13-12-9-5-4-8-11(12)10-6-2-1-3-7-10;2*1-5(2,3)4;/h9-11,16-21H,3-8,12-15H2,1-2H3;1-6,8-9H,13H2;2*1H3,(H,2,3,4);/q;-1;;;+2/p-2 |
InChI Key |
DDLMKPHKQUXHOT-UHFFFAOYSA-L |
Canonical SMILES |
COC1=C(C(=CC=C1)OC)C2=CC=CC=C2P(C3CCCCC3)C4CCCCC4.CS(=O)(=O)[O-].CS(=O)(=O)[O-].C1=CC=C([C-]=C1)C2=CC=CC=C2N.[Pd+2] |
Origin of Product |
United States |
Disclaimer and Information on In-Vitro Research Products
Please be aware that all articles and product information presented on BenchChem are intended solely for informational purposes. The products available for purchase on BenchChem are specifically designed for in-vitro studies, which are conducted outside of living organisms. In-vitro studies, derived from the Latin term "in glass," involve experiments performed in controlled laboratory settings using cells or tissues. It is important to note that these products are not categorized as medicines or drugs, and they have not received approval from the FDA for the prevention, treatment, or cure of any medical condition, ailment, or disease. We must emphasize that any form of bodily introduction of these products into humans or animals is strictly prohibited by law. It is essential to adhere to these guidelines to ensure compliance with legal and ethical standards in research and experimentation.