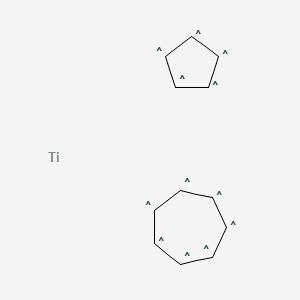
(|C7-cycloheptatrienyl)(eta5-cyclopentadienyl)titanium(ii)
- Click on QUICK INQUIRY to receive a quote from our team of experts.
- With the quality product at a COMPETITIVE price, you can focus more on your research.
Overview
Description
(|C7-cycloheptatrienyl)(eta5-cyclopentadienyl)titanium(ii), also known as Troticene, is a metallocene compound with the molecular formula C12H12Ti. It consists of a titanium atom sandwiched between a cycloheptatrienyl anion and a cyclopentadienyl anion. This compound is known for its unique structure and properties, making it a subject of interest in various fields of scientific research .
Preparation Methods
The synthesis of (|C7-cycloheptatrienyl)(eta5-cyclopentadienyl)titanium(ii) typically involves the reaction of titanium tetrachloride with cycloheptatriene and cyclopentadiene in the presence of a reducing agent. The reaction is carried out under an inert atmosphere to prevent oxidation. The product is then purified through recrystallization or sublimation to obtain a high-purity compound .
Chemical Reactions Analysis
(|C7-cycloheptatrienyl)(eta5-cyclopentadienyl)titanium(ii) undergoes various types of chemical reactions, including:
Oxidation: The compound can be oxidized to form titanium(IV) complexes.
Reduction: It can be reduced to form titanium(0) species.
Substitution: The cyclopentadienyl and cycloheptatrienyl ligands can be substituted with other ligands under appropriate conditions.
Common reagents used in these reactions include oxidizing agents like oxygen or hydrogen peroxide, reducing agents like lithium aluminum hydride, and various ligands for substitution reactions. The major products formed depend on the specific reaction conditions and reagents used .
Scientific Research Applications
(|C7-cycloheptatrienyl)(eta5-cyclopentadienyl)titanium(ii) has several scientific research applications, including:
Chemistry: It is used as a catalyst in various organic reactions, including polymerization and hydrogenation.
Biology: The compound is studied for its potential biological activity and interactions with biomolecules.
Medicine: Research is ongoing to explore its potential as a therapeutic agent due to its unique chemical properties.
Mechanism of Action
The mechanism of action of (|C7-cycloheptatrienyl)(eta5-cyclopentadienyl)titanium(ii) involves its ability to coordinate with various substrates through its titanium center. The cyclopentadienyl and cycloheptatrienyl ligands provide stability and facilitate the interaction with other molecules. The compound can participate in electron transfer processes, making it effective in catalytic applications .
Comparison with Similar Compounds
(|C7-cycloheptatrienyl)(eta5-cyclopentadienyl)titanium(ii) can be compared with other metallocene compounds such as ferrocene, nickelocene, and chromocene. Unlike these compounds, Troticene has a unique combination of cycloheptatrienyl and cyclopentadienyl ligands, which imparts distinct chemical and physical properties. This uniqueness makes it valuable for specific applications where other metallocenes may not be as effective .
Similar compounds include:
- Ferrocene (C10H10Fe)
- Nickelocene (C10H10Ni)
- Chromocene (C10H10Cr)
These compounds share the general metallocene structure but differ in the central metal atom and the specific ligands attached .
Properties
Molecular Formula |
C12H12Ti |
---|---|
Molecular Weight |
204.09 g/mol |
InChI |
InChI=1S/C7H7.C5H5.Ti/c1-2-4-6-7-5-3-1;1-2-4-5-3-1;/h1-7H;1-5H; |
InChI Key |
UGMSNGMGUIZFOA-UHFFFAOYSA-N |
Canonical SMILES |
[CH]1[CH][CH][CH][CH]1.[CH]1[CH][CH][CH][CH][CH][CH]1.[Ti] |
Origin of Product |
United States |
Disclaimer and Information on In-Vitro Research Products
Please be aware that all articles and product information presented on BenchChem are intended solely for informational purposes. The products available for purchase on BenchChem are specifically designed for in-vitro studies, which are conducted outside of living organisms. In-vitro studies, derived from the Latin term "in glass," involve experiments performed in controlled laboratory settings using cells or tissues. It is important to note that these products are not categorized as medicines or drugs, and they have not received approval from the FDA for the prevention, treatment, or cure of any medical condition, ailment, or disease. We must emphasize that any form of bodily introduction of these products into humans or animals is strictly prohibited by law. It is essential to adhere to these guidelines to ensure compliance with legal and ethical standards in research and experimentation.